
Only a select few organisms have found ways of harnessing energy external to their biological metabolism. For humans, this skill helped us flourish. But for how long?
A Brief History of Energy
The acquisition and consumption of energy is what drives every organism on the planet. Some species are more successful at securing energy than others, and that competition for a limited resource is what has driven evolution since the dawn of life on Earth. For most of Earth’s natural history, this process has maintained life in relative balance, but very rarely, a species adapts in such a unique and disruptive way that this balance is catastrophically interrupted.
About two and a half billion years ago, oceanic organisms found a remarkable new way of harnessing energy from their environment: oxygenic photosynthesis. This process led to an accumulation of oxygen in the atmosphere, and because there were no organisms to absorb this excess oxygen, it bonded with atmospheric methane and caused an anti-greenhouse effect that sent the planet into its longest ever period of glaciation (Sessions et al. 2009). These photosynthetic organisms, by finding a new way to harness energy, led the planet into a period of catastrophic climate change known as the Great Oxidation Event (Marshall 2015).

Humans are another uniquely disruptive organism. Over billions of years of Earth’s history, only a select few organisms have found ways of harnessing energy external to their biological metabolism. These creatures have used this excess energy to perform work beyond their physiological capacity (Burger 2011). Termites, for example, harness solar heat to cycle the air within their mounds, creating a solar-powered ventilation system (Griggs 2015). Gliding birds, such as albatrosses, harness the wind to travel long distances with very little work (Richardson et al. 2018).
Approximately half a million years ago, humans took this ability to a whole new level with perhaps the most important technological breakthrough in our history: the controlled use of fire. Since that point, we too have had the ability to harness extra-metabolic energy, and by using our unparalleled cognition, we have found applications for this energy that go well beyond the abilities of any other species in earth’s natural history.
For the first time, fire allowed us to extend our energy budget beyond our own biological constraints, significantly increasing the total work and demand for energy of which we are capable. Throughout the history of homo sapiens, extra-metabolic energy use has allowed societies to have a surplus of energy for meeting their most basic biological requirements. However, at no point has our evolutionary thirst for energy been satiated. Humans have constantly found new ways of utilizing this surplus energy by developing more energy intensive shelters, methods of food production, modes of transportation, and systems of manufacturing.
In recent years, evolutionarily speaking, the human capacity to harness extra-metabolic energy has accelerated. Just three centuries ago, the industrial revolution began introducing wave after wave of new energy demand by replacing physical labor with complex machines. This technological breakthrough created the necessary energy demand for the widespread extraction of fossil fuels: a concentrated underground energy source, the likes of which humans, nor any other species, had ever harnessed before. Over the last two centuries, fossil fuels have propelled us forward as a society, leading to unprecedented economic growth and technological innovation.
A Turning Tide

Objectively speaking, things have been going phenomenally well for the human species over the last half-million years. For many communities today, total metabolic demand for energy represents as little as 1% of overall energy use (Burger et al. 2017). All other species on the planet compete for a limited pool of biologically harvestable energy, known as the Net Primary Production of the planet (McDaniel and Borton 2002).
We have found a way to effectively remove ourselves from Net Primary Production constraints by utilizing sources of energy for which we have no competition.1 As a result we have multiplied in number and density, extended our life span, and temporarily freed ourselves from resource constraints. While famine and poor access to clean water persist in parts of the world, they are primarily a consequence of resource inequality rather than an environmental constraint on our species (Sen 1981).
We also have access to enormous quantities of surplus energy which we use to improve our comfort, develop culture, and expand our knowledge. This ability has allowed us to dominate the planet, and this trend will likely continue as we increasingly harness new energy sources such as solar, wind, and nuclear power.
However, after a half-million-year joyride, the human species is all of a sudden facing quite a few major roadblocks. We are rapidly approaching, or may have already crossed, several planetary constraints to our current growth trajectory. Unsustainable land use is leading to deforestation, desertification, and biodiversity loss, and the accumulation of waste products is threatening the chemical balance of the Earth.
Overcoming these obstacles will require that we radically change the way we think about energy. If we blindly follow our evolutionary propensity to consume ever increasing quantities of energy without regard for its impacts, we will soon reach a planetary tipping point from which our society may not recover. Right now, there is a global movement to shift away from fossil fuels and toward renewable sources of energy in order to staunch growing greenhouse gas emissions. This should certainly be a top environmental priority, but on its own will not put humanity on a sustainable path.
The source of our energy is only a piece of the puzzle. It is the nature of our energy use that must change, not just the resources from which we derive that energy. As McDaniel and Borton wrote in 2002, “A bulldozer running on solar generated hydrogen or fossil fuel accomplishes the same thing.” Both allow us to decimate the Amazon rainforest.
It is important to preface by saying that it is not the objective of this digest to subscribe to, or disseminate, a message of unavoidable catastrophe a la Thomas Malthus. On the contrary, the theory of extra-metabolic energy suggests that humans have a unique ability to expand the productivity of our planet for the wellbeing of both humans and nature. History has demonstrated time and time again that human innovation can successfully avert seemingly unavoidable crises, and our use of energy is fundamentally no different. However, in order to engineer our way out of the planetary crises caused by our unrestrained use of extra-metabolic energy, we must first recognize that our existing systems of production and waste are incompatible with a sustainable future.
A Future of Endless Energy
Humans are on the verge of a technological transition that could greatly increase our capacity to harness extra-metabolic energy. The rapidly falling costs of photovoltaics, wind turbines, and advanced storage technologies give us direct access to energy sources that will last as long as the Earth itself. Once we achieve this transition to renewable energy technologies, our species will no longer be limited by the available supply of organic fuels, ushering in a new era of renewable, affordable, and far more abundant energy.
History has shown us that surplus available energy is invariably used to grow the human population, expand economies, manipulate new landscapes, and produce new waste. When human access to energy of any kind—renewable or otherwise—increases, so too does our footprint on the planet. Rarely has our surplus energy been invested into supporting the overall health of the planet. It is an evolutionary imperative that this pattern of energy consumption is broken before our access to extra-metabolic energy from the sun and wind grows considerably. A truly sustainable path will require us to direct much of our surplus energy toward establishing a new ecological equilibrium via afforestation, reversing desertification, densifying land use, and reversing pollution.
Contextualizing Economic Growth
Energy is required as a primary factor in all economic production. As the second law of thermodynamics states: all transformation or movement of matter requires a minimum energy input. Therefore, because all production requires the transformation or movement of matter, all production demands a minimum energy input. Although modern economics insists that there are several primary factors including labor, land, and resources, some biophysical models of the economy, such as Bruce Hannon’s input-output model, derived from Nobel Laurate Wassily Leontief’s work, are built on the premise that energy is the only primary factor in production (Stern 2004).
Production is a method by which the human species can harness extra-metabolic energy, and economic growth is a representation of how efficiently and effectively a nation is utilizing available extra-metabolic energy. Today, one of the primary concerns of nations around the world is maintaining and accelerating economic growth; however, under mainstream frameworks, the perceived accrual of wealth from production only considers benefits to the human species.
We currently lack the institutional foresight to recognize that further increasing our environmental footprint is not actually in the long-term interest of our species. We are approaching a point in our evolutionary history where increasing our species’ footprint at the expense of ecosystems will lead to global ecological collapse, and in turn drastically worsen our own welfare as a species. We are already seeing evidence of approaching ecological thresholds with the desertification of grasslands, the loss of insect and coral biomass, and the thawing of the Arctic and Antarctic ice sheets (Moore 2018).
These environmental changes are, in time, going to have dramatic economic repercussions as coastal settlements are flooded, agricultural land is stressed, and fisheries collapse. It is time that we reprioritize our use of extra-metabolic energy so that global production improves the well-being of not only our own species, but also that of the entire biosphere.
More Energy, More Waste, More Land
All organisms produce waste. Life sustains itself by extracting energy from the environment in the most efficient way possible, and inevitably this leads to the production of biproducts from which an organism cannot derive a net gain in energy. Within a healthy ecosystem, these biproducts simply become the energy input for other specialized organisms. However, these ecosystem relationships emerge concurrently over millions of years of evolution.
In just a few thousand years, extra-metabolic use of concentrated energy sources has allowed humans to mine raw materials, transform them into useful ingredients, and then dispose of them when they no longer provide value. Much of this displaced material is toxic, disrupts the planet’s chemical balance, and cannot be decomposed by other organisms. During the Great Oxidation Event, oxygen was the waste product, and the inability of other organisms to absorb it led to catastrophic global disruptions. It took millions of years for a new oxygen-inclusive equilibrium to establish itself.2
A warming planet is just one particularly scary side-effect of our unrestrained use of extra-metabolic energy to produce waste; specifically, the pollution of the planet’s atmosphere with billions of tons of carbon dioxide. However, humans also dump millions of tons of other compounds and chemicals into our air, soil, and water every year. More than 140,000 of these chemicals are man-made and would never have occurred in nature if it had not been for our ability to synthesize them with extra-metabolic energy.
Today, public enemy #1 is carbon dioxide, but at some point, we are also going to need to address the plastics, nuclear waste, fertilizers, endocrine disrupting compounds, and heavy metals that are contaminating the planet every day. Climate change is the first existential pollution crisis to threaten our species, but it certainty will not be the last. Pollution of all kinds is the result of how humans have chosen to use the surplus energy that we have unique access to. A transition to renewable energy resources may successfully reduce waste CO2, but it will also provide us with the opportunity to increase our capacity for energy use, and in doing so, hasten the production of other pollutants unless we radically change the way we use the energy that is available to us.
Our ever-increasing land-use is also a symptom of our energy addiction. Agriculture and resource extraction have been two of our most successful innovations in the extra-metabolic use of energy, but they have also amplified our ecological footprint. Since the 1700s, the percentage of ice-free land considered “wild,” or uninhabited and hosting no significant human land-use, has declined from 50% to 25% (Ellis et al. 2010). This expansion in our land-use footprint has led to widespread deforestation and such a severe loss of biodiversity that many scientists now suggest that we are rapidly approaching a sixth mass extinction event on par with the Cretaceous–Paleogene extinction event that wiped out 76% of all species 66 million years ago (Gibbons 2011).
Humans are the most intelligent and innovative species ever to exist on Earth, but we still rely heavily on ecosystems to provide us with clean water, oxygen, healthy soil, and food. If we want future generations to thrive, we need to halt the frightening pace of biodiversity loss and the shrinking of healthy ecosystems.
Internalizing Externalities
In many respects, the transition to renewables is a test of whether or not our species is able to reprioritize its extra-metabolic energy use. If we are successful in internalizing the planetary cost of carbon emissions into our economic systems, it then seems achievable to successfully internalize the cost of other pollutants. However, if we fail to incorporate planetary constraints into our economic principles, and instead allow technological innovation and existing market competition to drive us toward increasingly affordable renewable energy, we could find ourselves in a fatal situation of having vast renewable energy access and no institutionalized environmental principles with which to limit our use of it. Our civilization, and the future health of the planet, depends as much on how we transition to renewables, as it does on when that transition takes place.
In recent years, solar and wind generated electricity has become increasingly cost-competitive with conventional fossil fuel power. Storage technologies such as lithium ion batteries remain expensive but are also falling sharply in price. At the same time, extraction of the planet’s fossil fuel resources will eventually peak, and fossil fuel powered infrastructure will retire. It will not be long before the economic argument for investment in fossil fuels will be unconvincing to even the staunchest climate change deniers.
In recent years, solar and wind generated electricity has become increasingly cost-competitive with conventional fossil fuel power. Storage technologies such as lithium ion batteries remain expensive but are also falling sharply in price. At the same time, extraction of the planet’s fossil fuel resources will eventually peak, and fossil fuel powered infrastructure will retire. It will not be long before the economic argument for investment in fossil fuels will be unconvincing to even the staunchest climate change deniers.
We are so preoccupied with the goal of reaching a carbon-free future, that the inevitability of an eventual transition to renewable energy sources is seen as a reason for optimism. It could not, unfortunately, be farther from the case. Allowing existing market competition to drive the transition to 100% renewables will not be a victory, even if it does meet the goals of the Paris Agreement. A transition driven by existing economic frameworks will address a single pollution crisis just by the luck of technological innovation and resource depletion, but it will not fundamentally change our treatment of the planet nor our relationship with extra-metabolic energy. Instead it will remove existing constraints on energy production and hasten the use of that energy for self-serving and environmental detrimental purposes.
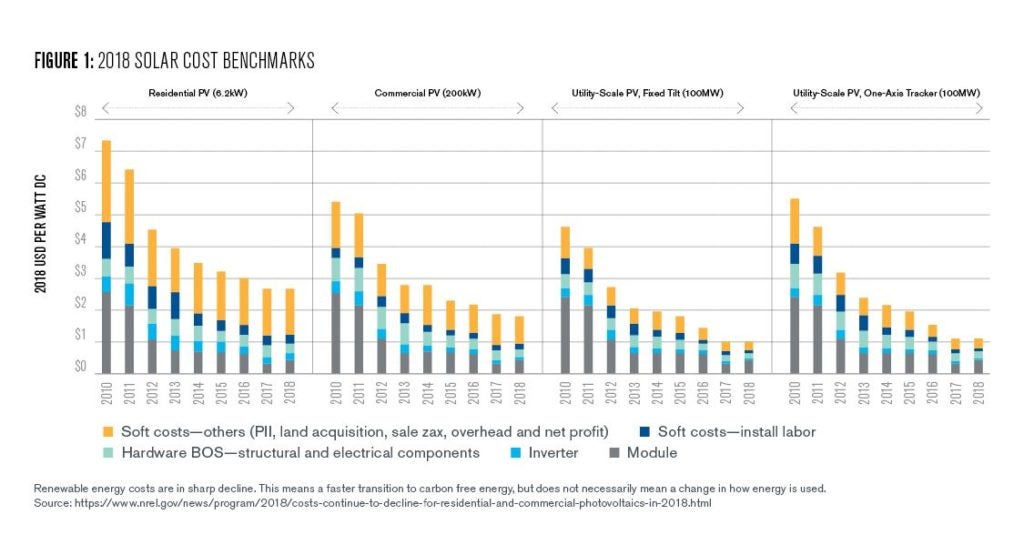
In the face of climate change, one often extolled strategy to reduce CO2 emissions is to institute a method of carbon pricing. For every ton of carbon that a producer emits, they must pay a determined price—either in the form of a tax or credit—that reflects the external cost to the planet of that ton of carbon. The idea of a carbon price is to introduce a negative value of carbon pollution into mainstream economic models, reducing the economic drive to pollute.
Policy researchers, legislators, and climate scientists have been pushing for a price on carbon for years, but in countries around the world these calls have been met with significant pushback. Last fall, the world watched as French citizens protested a carbon tax on diesel, and in Washington state, a carbon tax bill was once again defeated, thanks in part to oil companies spending more than $30 million to fight it (Gillis 2018).
Opponents of a carbon pricing structure argue that it will hurt the economy or will impose unacceptable costs on consumers, demonstrating society’s persistent denial of the true relationship between the health of the planet and our health as a species. Accepting the need to internalize environmental costs into our economic system does not require us to accept an end to economic growth. On the contrary, in so far as the economy is a representation of human wellbeing, it is an evolutionary imperative that we adapt as a species to improve human and ecological wellbeing in tandem. The human capacity to harness extra-metabolic energy means that we have more than enough capacity as a society to invest in both social and economic development as well as ecological productivity and resilience. The threat to long-term economic growth is short-sightedness, not insufficient capacity or resources.
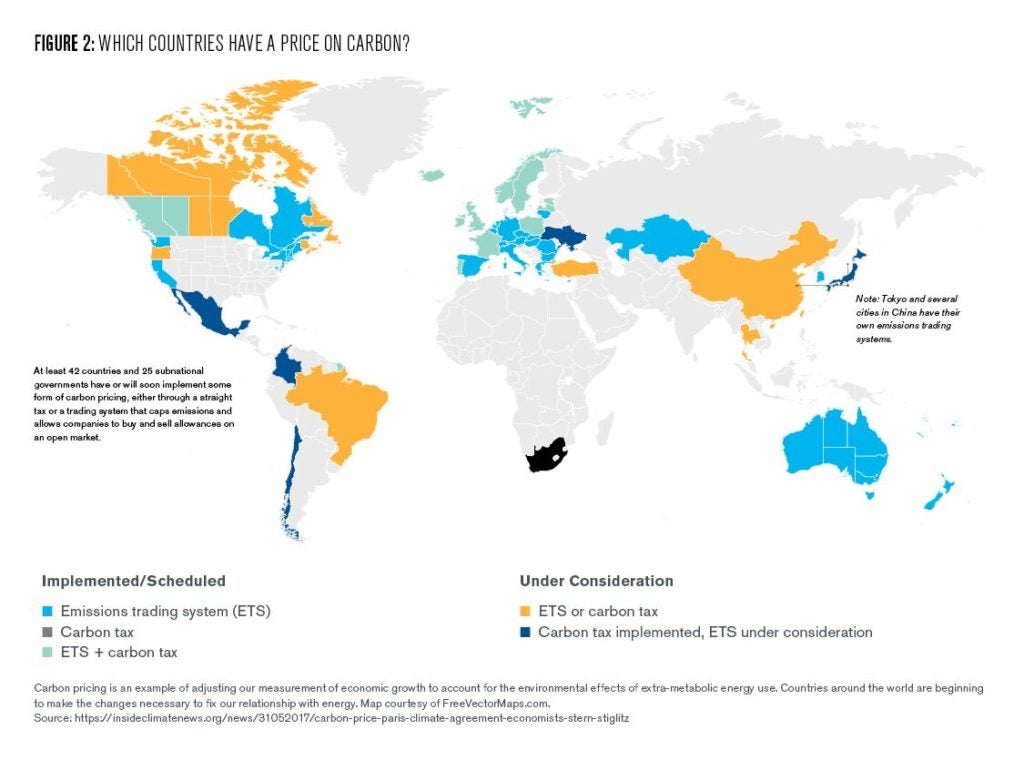
The concept of internalizing externalities is fundamentally important to managing our current CO2 crisis, and is the first conception of a systemic change that must be adopted by global markets and communities. CO2 is just one of hundreds of poorly regulated pollutants with which humanity will eventually have to grapple if we continue to have access to considerable extra-metabolic energy. Managing carbon emissions is currently the top priority, but eventually we are going to need a system that internalizes the planetary cost of all waste and displaced ecosystems.
In order to build a truly sustainable society, external costs of our growing ecological footprint have to be incorporated just as robustly and universally as are the benefits of extra-metabolic energy use. Our current system of measuring economic growth and wealth is a one-sided equation. It recognizes the benefits imparted to the individual and to the human species by the use of extra-metabolic energy, but it largely ignores the planetary impacts of our energy use. Only by internalizing the costs to the planet will humans be able to reprioritize our extra-metabolic use of energy, and in doing so prevent ecological and societal catastrophe.
Conclusion
Humans are fundamentally unlike any other living organism that has ever existed. In the three and a half billion year history of life on Earth, we are the only species who has developed technologically advanced methods of using energy independent of our metabolic capacity. This unique ability is at the core of what allows our modern society to function.
Agricultural production, manufacturing, computing, and transportation are all made possible by surplus energy that we have harnessed from our environment. We now live in a world where our ability to harness energy threatens our society and the health of the planet. Emissions from fossil fuels are heating the planet, waste products and pollution are choking ecosystems, and our land-use is pushing other species to the brink of extinction.
Photovoltaic cells, wind turbines, and battery storage technology hold the key to solving climate change, the planet’s most pressing pollution crisis. However, these same technologies have the potential to massively expand the quantity of energy available for human use. Unless we fundamentally reassess our energy use priorities, climate change will be just the first of many existential crises we bring upon ourselves and the planet. If we are going to respond successfully to climate change and prevent other waste crises in the future, we need to rework our economic models so that they successfully prioritize using our extra-metabolic energy for waste reduction and land preservation rather than waste production and ecosystem exploitation.
Oscar Serpell
Deputy DirectorOscar Serpell oversees all student programming, alumni engagement, faculty and student grants, and visiting scholars. He is also a researcher, writer, and policy analyst working on research initiatives with students and Center partners.
Burger, Joseph et al. 2017. Extra-metabolic energy use and the rise in human hyper-density. Scientific Reports 7:43869 https://bit.ly/2RqGL36
Burger, Oskar et al. 2011. Industrial Energy Use and the Human Life History. Scientific Reports 1:1. https://www.nature.com/articles/srep00056
Ellis, Erle et al. Anthropogenic transformation of the biomes, 1700 to 2000. Global Ecology and Biogeography. 19, 589–606 https://bit.ly/2MTTJYB
Gibbons, Ann. 2011. Are We in the Middle of a Sixth Mass Extinction? Science Magazine. https://bit.ly/2ZDWuif
Gillis, Justin. 2018. Forget the Carbon Tax for Now. New York Times Opinion. https://nyti.ms/2Q5NtJh
Griggs, Mary Beth. 2015. Termites Engineer Solar-Powered Ventilation Into Their Mounds. Popular Science. https://bit.ly/2RkeFGr
Marshall, Michael. 2015. The Event That Transformed Earth. BBC Earth. https://bbc.in/1IxPDeb
McDaniel, Carl and Borton, David. 2002. Increased Human Energy Use Causes Biological Diversity Loss and Undermines Prospects for Sustainability. Bioscience. 2:10 https://bit.ly/2XlzaYQ
Moore, John C. Predicting Tipping Points in Complex Environmental Systems. PNAS, vol. 115 No. 4 https://bit.ly/2Kn0gsr
Richardson, Philip L. et al. 2018. Flight Speed and Performance of the Wandering Albatross with Respect to Wind. Movement Ecology 6:3. https://bit.ly/2FeL2BE
Sen, Amartya. 1981. Poverty and Famines: an Essay on Entitlement and Deprivation. Clerendon Press. https://bit.ly/2Fhi7gj
Sessions, Alex et al. 2009. The Continuing Puzzle of the Great Oxidation Event. Current Biology vol.19 iss. 14. https://bit.ly/2F2GUW9
Stern, David. 2004. Economic Growth and Energy. Encyclopedia of Energy, Volume 2. http://sterndavidi.com/Publications/Growth.pdf
- One could argue that pests such as mice, rats, locusts, and crows represent a form of competition for our food resources but, at least for the time being, this competition is successfully controlled through the use of pesticides and management practices. [↩]
- Perhaps in millions of years life will evolve to consume our synthetic waste, but that is not something we can rely upon in the here and now. [↩]