Introduction
The electricity grid has similarities to the human body, an interrelated system of muscles and organs (i.e. power generators), arteries (i.e. transmission lines) and veins and capillaries (i.e. distribution lines) all working and pulsing together. Electrical current on the grid moves cyclically, ideally at a specific frequency, kind of like a heartbeat. Electric system operators need to monitor the grid’s heartbeat and other factors to maintain its health in order to keep the lights on. Even slight deviations from the normal pulse can cause major problems if not quickly detected and corrected.
Until now, grid operators have used older technology to monitor the grid’s heartbeat. However, the grid has a fast pulse and the old technology can’t keep up. This is especially true as electricity system needs change in response to threats such as extreme weather and innovations like renewable energy, demand response, and energy storage. Synchrophasor technology has the potential to more quickly and accurately measure and monitor the grid, making it more resilient, reliable, and capable of integrating cleaner energy resources.
A recent nation-wide push to deploy this technology on the transmission system has established an infrastructure framework that is improving grid operators’ understanding of electricity system health. Not only does Pennsylvania play a significant role in this national transmission system effort, Philadelphia is also hosting a first-ever pilot to test the technology at the distribution system level.
This digest provides an overview of current power system challenges, synchrophasor technology basics and efforts to deploy the technology. The purpose of this digest is to explain the technology, its applications, and potentials to a non-engineering audience.
Modern Power System Challenges
Functioning electrical system infrastructure—such as power generation, transmission and distribution equipment—is critical to modern life. Power systems must maintain the balance between power supply and demand and ensure the system can resiliently and reliably deliver power to customers. Achieving these goals is becoming more complex as aging power systems must integrate new technologies with unique operational profiles (e.g. renewable energy that has variable power output, energy storage, distributed generation), maintain performance in more extreme weather conditions, defend against cybersecurity threats, and accommodate changing load (demand for power) and generation profiles. These and other factors combine to complicate electric system operations, especially in coordinating the production and movement of power through long-range transmission and shorter-distance distribution infrastructure.
In response to these and other challenges, such as the August 2003 blackout in the northeast U.S., the North American Electricity Reliability Corporation (NERC) issued a report in 2008 calling for a number of “minimum acceptable capabilities and best practices for real-time tools necessary to ensure reliable electric system operation and reliability coordination.”1 NERC is the national electric reliability organization that develops electric reliability standards, monitors enforcement and compliance with those standards, analyzes the adequacy of resources available to maintain reliability, and provides technical training and assistance to power system operators.
NERC determined that synchrophasor technology can meet many of the 2008 report’s demands to improve real-time tools as well as the situational awareness of grid operators, while also improving the grid operator’s ability to assess both system security and the system’s ability to handle contingencies.
Why Synchrophasor Technology?
Traditionally, supervisory control and data acquisition (SCADA) systems have been used to monitor and control power systems. SCADA systems include hardware and software that collect, transmit, store and communicate data. SCADA systems obtain data every 2 to 4 seconds from meters, transducers, intelligent electronic devices (IEDs), and other hardware devices. Data collected and transmitted can include the status of breakers and switches, real and reactive power flows, and voltage magnitude. SCADA systems begin to fail when the electricity system is changing quickly. In general terms, this failure is due to the SCADA systems being too slow to accurately capture the faster-moving electrical system changes.
Synchrophasor technology provides more data with better accuracy. Mailbox-sized monitoring devices called phasor measurement units (PMUs) provide high-resolution information. Specifically, they sample grid conditions one hundred times per second and convert these data into phasor values—a mathematical representation of the magnitude and phase angle of electric voltage or current. From these samples, approximately 30 phasor values are delivered per second, which are then synchronized via time-stamping from global positioning systems (GPS), converting the phasor values into synchrophasors. Synchrophasor data from PMU’s installed throughout the electric power system are sent through communication networks to various phasor data concentrators (PDC) that collect and time-align the data. The time stamping allows data collected from multiple areas to be accurately combined together to create a consistent operational picture of the entire grid.
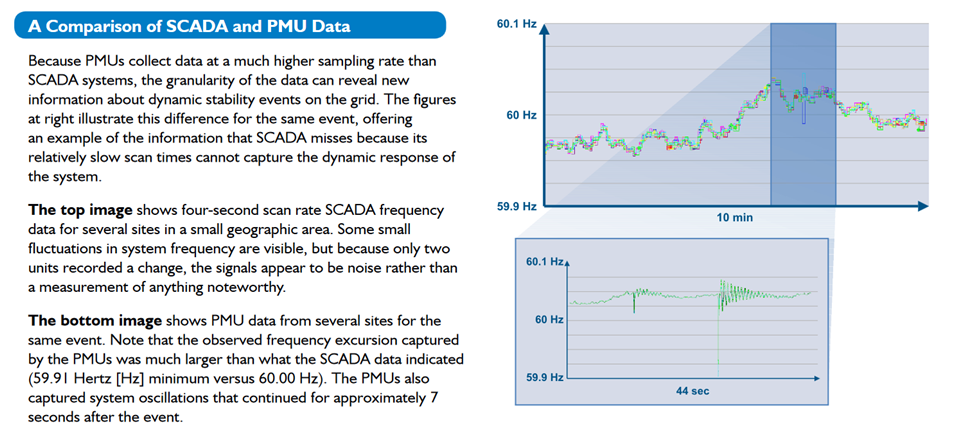
According to the U.S. DOE, synchrophasor technology has a variety of potential operational benefits, generally broken down into “real-time” capabilities and “study mode/planning” capabilities. Examples of these include:2
- Increased system reliability. This can occur as operators better monitor conditions to prevent outages, diagnose failing equipment, enable faster re-energizing of out-of-service transmission lines, determine real-time operational limitations of the grid and enable evaluation of actual system measurements rather than mathematical estimates.
- Increased renewable energy integration. This can be enabled through synchrophasor technology, as PMUs allow for real-time monitoring of system voltage and frequency to determine the grid impacts of intermittent renewable generation, enable better modeling of renewable energy generation, and allow for better grid management of these resources by understanding individual plant control systems.
- Improved organizational efficiency. This can occur by reducing the time (up to 75% reduction), effort and costs associated with analyzing system disturbances, validating models, and assessing the health of the grid.
National Deployment Success and Utilization Challenges
The U.S. DOE recently published a report detailing the results of a significant, national synchrophasor deployment effort.3 The 2009 American Recovery and Reinvestment Act (ARRA) provided funding for the U.S. Department of Energy to competitively award to grid modernization projects. The U.S. DOE selected 13 entities to carry out 13 synchrophasor-related projects. The total cost of these projects was about $358 million, of which 56 percent was privately funded and 43 percent ARRA supported.
Before this public-private effort, there were only 166 research-grade, networked PMUs in North America. By 2015, the ARRA-supported effort resulted in installation of an additional 1,380 networked PMUs and 226 PDCs. With the addition of 150 new PMU’s that were installed using 100 percent private funds, there are now over 1,700 PMU on the North American grid, providing near 100 percent visibility of the U.S. high-voltage transmission system that serves approximately 88 percent of the total U.S. electricity load. These synchrophasor investments have been concentrated on the transmission system, but as this digest will explore later, there could be value on the distribution system as well.
Now that the majority of the PMU and PDC infrastructure is in place, it is up to the system operators to take full advantage of the technology’s potential capabilities. However, there are implementation barriers preventing this technology from reaching its full potential. Barriers to the operational use of the installed synchrophasor assets generally fall into two categories: procedures and data sharing. Each system operator has unique operational protocols governing its various grid operations. To integrate synchrophasor data, operators must develop, test, and validate new operational procedures—a time consuming task. Of the 13 entities that received ARRA funding for synchrophasors, only five have formally implemented these new procedures.
The extent to which the PMU data can create a complete picture of the grid depends on data sharing among the various operators that run grids covering different geographic areas. Enabling data sharing among different grid operators requires development of a wide range of applications to handle these “big-data” needs from a wide variety of users, each with unique systems and requirements. The ARRA-funded effort has increased data sharing, but only the western portion (Western Interconnection Synchrophasor Project) of the U.S. grid has implemented an interconnection-wide, data-sharing platform.
Data quality concerns around accuracy and timeliness have been another challenge with synchrophasor technology in the past. DOE reports that data quality and trustworthiness improved greatly as a result of the best practice and coordination efforts supported by the ARRA projects.
U.S. DOE continues to support synchrophasor technology improvements through strategic grants. For example, in 2013 the agency made $9 million in funding for software development to support and improve PMU data utilization4 , and in March, 2016 the agency announced a $5 million funding opportunity to support PMU software advancement for reliability and asset management.5
Pennsylvania’s Synchrophasor Projects
Pennsylvania happens to be home to two significant synchrophasor projects. One is part of the ARRA-funded national effort that is being implemented by PJM interconnection, the grid operator covering 13 Mid-Atlantic States and the District of Columbia. The second is a project at the Philadelphia Naval Yard testing PMU technology on the distribution system.
PJM Interconnection’s Large-Scale Deployment
PJM Interconnection worked with 12 transmission system owners in its territory, leveraging ARRA funds to deploy 301 PMUs and 23 PDCs across its system, providing coverage over 10 states.6 The project, which also included development of a synchrophasor communications network, cost over $27 million with the federal cost share at a little over $13.5 million. The objectives of the project were to improve electric system reliability, wide-area observability, and event detection and analysis.
In addition to installing the PMU and PDC hardware, PJM deployed communications infrastructure, built systems to allow data visualization, applications to process and leverage the incoming data (e.g. disturbance analysis, frequency, angle and oscillation monitoring), and developed a cyber-security plan to ensure data safety. PJM has also begun to incorporate synchrophasor data into its process of operations and event analysis (i.e. procedures) and has begun to send data with some of its neighboring grid operators (i.e. data sharing).
PJM developed a Phasor Data Quality Task Force to resolve issues and reported that 91 percent of the data was acceptable, with the overall trend moving towards the 0.2 percent error rate.7 Reasons for data error were attributable to server overloads, loose cables, loss of telecommunications connections, GPS clock issues, and other factors.
Moving forward, PJM intends to continue its efforts to fully leverage the synchrophasor investments by incorporating synchrophasor data and visualization capabilities into their control room, developing a power plant operations model validation tool using PMU data, developing a PMU training schedule for grid operators, and other potential activities.
The Navy Yard’s Micro-PMU Pilot
Once a naval base, “The Navy Yard” is situated on League Island near downtown Philadelphia. The sprawling campus has undergone a long-term redevelopment effort into a business and industry hub.
The U.S. DOE chose Philadelphia’s Navy Yard as the site of a new study to test micro-PMU technology on the local distribution system.8 The project involves the Philadelphia Industrial Development Corporation (PIDC) that manages the Navy Yard’s grid, the Grid Integration Group at Lawrence Berkeley National Lab, U.S. DOE, PECO (the local electric utility), and several other partners.
The Yard has its own electricity grid that is not regulated by the state utility commission. The un-regulated nature of the Yard’s grid makes it an ideal location for testing new grid technologies. In addition, the Navy Yard has embarked on an ambitious effort to modernize its energy systems and create a microgrid, creating further potential to test and integrate advanced technologies.
In 2013, the Navy Yard released a 10-year energy master plan to accompany the Yard’s broader real estate development master plan update.9 .10 The energy master plan recognized the need for a reliable, modernized energy system to accommodate the predicted 200 percent growth in tenant energy needs as the island-property redevelops.11 The energy master plan included five focus areas: 1) smart grid infrastructure, 2) business model development, 3) building owner (end-user) opportunities, 4) test bedding outreach and protocols, and 5) carbon reduction and sustainability. Some of the energy master plan components include deploying advanced metering infrastructure, developing a grid network operating center, establishing demand response and energy efficiency programs, enabling distribution system automation, expanding the grid, installing a variety of generation assets, and developing a new utility business model.
As part of the 2009 ARRA, Penn State University received over $5 million in funding from the U.S. DOE (with additional funding from other sources) to develop a Grid Smart Training Application and Resources (GridStar) Center at the Navy Yard.12 The GridStar Center is a research and education facility for smart grid technologies that includes workforce training programs and a microgrid demonstration facility called the Smart Grid Experience Center. The Smart Grid Experience Center includes electric vehicle charging, battery storage, solar PV, home automation, energy management applications, smart meter infrastructure, and other components.
In 2014, Alstom was awarded $1.2 million by the U.S. DOE to improve grid resiliency at the Navy Yard through the installation of advanced microgrid technology.13 (Note: In November 2015, General Electric acquired Alstom’s power- and grid-related business units.) The Alstom project aims to address grid resiliency and the growth in distributed energy resources (e.g. storage, renewables, demand response, small scale generation) through development of a prototype microgrid controller systems (a combination of energy management and substation automation technology). The microgrid controller system will enable specific grid functionalities, such as microgrid islanding, synchronization and reconnection, protection, voltage frequency, power quality management and system resiliency. For example, the project should enable the Navy Yard campus to operate independently (islanding) from the main power grid in the event of a wide-scale power outage and safely synchronize and reconnect to the system when appropriate. In addition, Alstom, Penn State University, and other partners developed a first-of-its-kind, global microgrid Center of Excellence at the Navy Yard aimed at advancing development of microgrid technologies.14 These activities all set the stage for the Navy Yard to be an attractive location to test micro-PMU technology at the distribution level.
In 2012, ARPA-e provided $4 million to the University of California Berkeley to partner with Lawrence Berkeley National Labs and other partners to develop the micro-PMU technology for distribution-level use, and the technology has been deployed in over 20 locations in the U.S.15 However, the micro-PMU project is apparently the first of its kind, piloting the deployment of micro-PMUs on a functional microgrid with control integration.16 According to Lawrence Berkeley National Labs, the project will allow for advanced sensor integration and data analytics based on deployment of four micro-PMU operating in real system conditions. This will provide an opportunity to demonstrate advanced analytics and algorithms in an operational environment. The Navy Yard’s micro-PMU project is scheduled to begin once certain portions of the Yard’s current grid modernization efforts are completed, scheduled for June 2016. For example, installation of certain equipment that will allow for segments of the grid to be isolated from other customers, to enable testing and experiments without service interruptions.
The broad research question asks if deployment of synchrophasor technology on the distribution system can enhance power flow planning, system control, security, and resiliency for the next generation electricity system—an electricity system with data and analytic needs that are becoming more complex as distributed energy generation penetration increases. Synchrophasor technology has demonstrated value for the transmission system, but the distribution system has comparatively more components that create unknowns and potential for error. Test deployment of micro-PMUs can reveal unknowns, validate data and models, and give distribution planners more confidence about distribution level value.
Conclusion
Synchrophasor technology has the ability to give grid operators more timely and accurate information about the health of the grid, helping to prevent outages, better integrate new energy technologies, and save money by reducing planning and operational costs. It is one of the tangible technologies that could make the grid smarter. Major national efforts have resulted in a large-scale infrastructure buildout of synchrophasor technology on the bulk power transmission system and ongoing federal efforts aim to leverage that infrastructure investment. Pennsylvania is host to transmission-level PMU deployment, and has plans to house the first pilot to test micro-PMU technology on an operating distribution system.
Leveraging the success of the national transmission-level deployment project and demonstrating the technology’s value to the distribution system will help realize the grid of the future, a future where the system must be more resilient and flexible while tolerating technology and customer transformation.
Feature Image: “Courtesy of the North American SynchroPhasor Initiative (www.naspi.org) and the U.S. Department of Energy.”
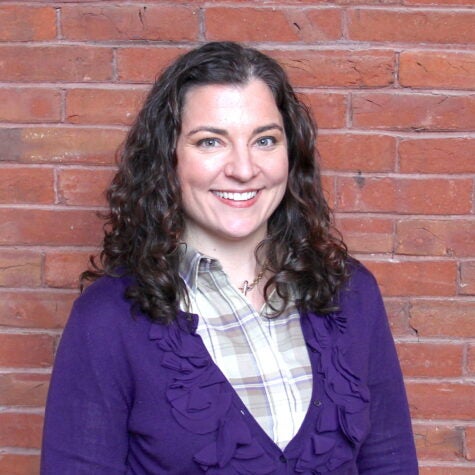
Christina Simeone
Donolo, Marcos A., “Advantages of Synchrophasor Measurements Over SCADA Measurements for Power System State Estimation,” Schweitzer Engineering Laboratories, Inc, 2006, https://cdn.selinc.com/assets/Literature/Publications/Application%20Note…
Kumar, Dr. Jayant, “Alstom Microgrid System for Philadelphia Navy Yard,” presentation for NREL Advanced Grid Technology Workshop Series, July 7-9, 2015, http://www.nrel.gov/esi/pdfs/agct_day3_kumar.pdf
North American Electric Reliability Corporation (NERC), “Real-Time Application of Synchrophasors for Improving Reliability”, October 10, 2010, http://www.nerc.com/docs/oc/rapirtf/RAPIR%20final%20101710.pdf
U.S. DOE, “Synchrophasor Technology and Renewables Integration,” NASPI Technical Workshop, NASPI Synchrophasor Technical Report, June 7, 2012, http://energy.gov/sites/prod/files/2014/07/f17/NASPI-NREL-TechRpt-Renewa…
Figure 1 Image Courtesy of:
U.S. DOE’s “Summary of the North American SynchroPhasor Initiative (NASPI) Activity Area,” June 2012, http://energy.gov/sites/prod/files/North%20American%20Synchrophasor%20In…
- Real-Time Tools Best Practices Task Force, “NERC Real-Time Tools Survey Analysis and Recommendations, Final Report,” March 13, 2008, http://www.nerc.com/comm/OC/Pages/Real-time-Tools-Best-Practices-Task-Fo… [↩]
- U.S. Department of Energy, “Advancement of Synchrophasor Technology: In projects funded by the American Recovery and Reinvestment Act of 2009,” March 2016, available at https://www.smartgrid.gov/files/20160320_Synchrophasor_Report.pdf [↩]
- IBID, U.S. DOE (March 2016) [↩]
- U.S. DOE, “Energy Department Announces Funding to Provide Better Visibility into the Health of the Nation’s Electric Grid,” September 26, 2013, DE-FOA-0000970, http://energy.gov/articles/energy-department-announces-funding-provide-better-visibility-health-nation-s-electric-grid [↩]
- U.S. DOE, “Increasing Reliability of the Nation’s Power Grid through Greater Visibility,” March 22, 2016, DE-FOA-0001492, http://energy.gov/oe/articles/increasing-reliability-nation-s-power-grid-through-greater-visibility [↩]
- U.S. DOE, “PJM Interconnection Synchrophasor Technology Deployment Project: Final Project Description,” Smart Grid Investment Grant of 2009 ARRA, June 2015, https://www.smartgrid.gov/files/PJM_Updated_Project_Description.pdf [↩]
- Liu, Jing, “The Deployment of the PJM Synchrophasor Project and Lessons Learned,” PJM Interconnection, presentation to the IEEE Innovative Smart Grid Technologies Conference, February 20, 2014,
http://sites.ieee.org/isgt2014/files/2014/03/Day2_Panel1B_Liu.pdf [↩] - The Yard Blog, “U.S. Department of Energy Selects the Navy Yard as the Location for Testing Micro-PMU Technology,”, March 29, 2016, http://www.navyyard.org/theyardblog/2016/03/29/u-s-department-of-energy-selects-the-navy-yard-as-a-location-for-testing-micro-pmu-technology/ [↩]
- The Burns Group, “The Navy Yard: Energy Master Plan,” February 2013,
https://db.tt/qo01YX6P [↩] - Robert A.M. Stern Architects, “The Navy Yard Master Plan 2013 Update,
http://www.navyyard.org/master-plan-2013/ [↩] - Burns Engineering Inc, “Smart Micro-grid Energy Master Plan for the Philadelphia Navy Yard,” Consulting Specifying Engineer (CSE) Magazine, August 14, 2014,
http://www.csemag.com/single-article/burns-engineering-inc-smart-micro-grid-energy-master-plan-for-the-philadelphia-navy-yard/2c0672091bf0c4a0919a7c593204be2f.html
[↩] - More information about Penn State’s GridStar program can be found at
https://smartenergyacademy.psu.edu/gridstar/ [↩] - Alstom Website, “Alstom received $1.2M DOE funding to advance Philadelphia microgrid project,” October 2014,
http://www.alstom.com/press-centre/2014/10/alstom-receives-12m-doe-funding-to-advance-philadelphia-microgrid-project-/ [↩] - Penn State University Website, “Penn State partners with Alstom to establish Microgrid Center of Excellence,” June 2015,
http://news.psu.edu/story/359626/2015/06/03/impact/penn-state-partners-alstom-establish-microgrid-center-excellence [↩] - Lawrence Berkeley Livermore Lab Website,“Micro-Synchrophasors for Distribution Systems,”
https://distgrid.lbl.gov/project/micro-synchrophasors-distribution-systems [↩] - Lawrence Berkeley Livermore Lab Website, ”Data-Driven Approach to Monitoring and Control of Distribution System Assets in Microgrid, Using Micro-PMU Technology,”
https://distgrid.lbl.gov/project/data-driven-approach-monitoring-and-0 [↩]