Should Maximum Contingency Fuel be Regulated for U.S. Civil Aviation?
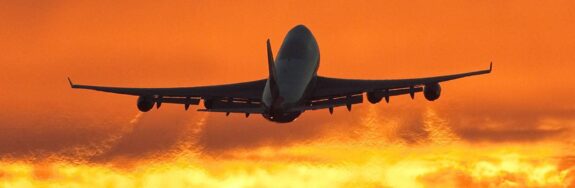
Air transportation contributes 8% of transportation greenhouse gas emissions in the U.S. and 10.6% of transportation emissions globally. As these shares are both expected to increase as incomes rise and the U.S. recovers from the 2008 recession, airlines and Air Navigation Service Providers (such as the Federal Aviation Administration) are united in their goal to reduce fuel consumption. Given the difficulty, cost, and long timeline of reducing aviation fuel and GHG emissions with new procedures, new technologies, and alternative fuels, airlines are turning to a simple and well-tested method to reduce fuel consumption: reducing aircraft weight from eliminating non-essential items on board a flight.
What if one of these non-essential items was fuel? Surely all fuel on a flight cannot be considered non-essential, but it is possible that a portion of the fuel on a flight, loaded in a discretionary manner for contingencies such as unexpected weather, might be over and above what might be needed for foreseeable contingencies. Beyond fuel required to complete a flight, or “mission fuel,” the FAA regulates (though U.S. Federal Aviation Regulations 14 C.F.R. § 91) the amount of reserve fuel that must be added for contingencies; for example, a flight may have to hold above an airport before landing because of congestion, thus requiring extra fuel. It is, however, possible for airlines to add fuel beyond the mandated reserve fuel. In fact, it is common for airlines to load fuel for contingencies guided by the amount of extra fuel, compared to the planned mission fuel that was needed in the past year. Airline dispatchers load this “contingency fuel” guided by what is termed “Statistical Contingency Fuel.” For example, a flight with the quantity of fuel for contingencies uplifted equal to SCF99 would have been able to land without dipping into any reserve fuel for 99% of flights during the past year. Airline dispatchers might also add enough fuel to travel to an alternate airport; in poor weather conditions this is required by the regulations, and in good weather conditions it is not required and thus might be added as a form of contingency fuel.
One might ask, isn’t reducing contingency fuel loads dangerous? It is not clear as it is unknown how much contingency fuel airlines are loading, and if it is higher or lower than the statically conservative buffer of SCF. To be clear, a flight that runs low on fuel while enroute to a destination would not simply fall out of the sky but rather divert to an alternative airport. Diversions are not inherently dangerous but they are costly. Airlines load discretionary fuel to reduce the probability that a flight will need to divert to an alternate airport, which costs approximately $25,000 in direct operating costs. When loading discretionary fuel, airlines must make the complex trade-off between the probability of diverting and the absolute cost of carrying contingency fuel.
What is the real benefit of reducing contingency fuel uplift? In short, an aircraft burns fuel to carry weight; excess fuel is excess weight. To find the exact amount of fuel that is burned due to carrying an extra pound of weight – or contingency fuel – we estimated cost to carry factors for each aircraft capturing the fuel consumed to carry a unit of fuel one mile (in lb/lb-mile). Cost to carry factors allow us to convert contingency fuel loaded into fuel burned. Using a large quantity of simulated gate-to-gate aircraft fuel consumption data from a state-of-the-practice aircraft performance analysis software, we model fuel consumption for a range of aircraft mass values that capture different amounts of fuel uplift and distances, all for a range of aircraft types. The results are cost to carry factors that relate a pound of fuel uplifted onto a flight to extra fuel consumed; the empirical results tell us that, for example, most aircraft burn an extra pound of fuel just to carry 10 pounds of fuel a distance of 2,000 miles.
Next we estimate the fuel burned and the greenhouse gas emissions released from a flight carrying fuel that is not consumed on a flight. A major U.S. network airline provided an individual flight dataset for most of their flown flights within the CONUS (roughly 570,000 after removing spurious observations) between June 1, 2012 and May 31, 2013. The final dataset we assembled has, for each flight:
- Gate-to-gate fuel burn
- Fuel uplift in all categories:
- Required fuel: mission fuel and required reserve fuel (including 45 minute hold and fuel to travel to a required alternate airport)
- Fuel that is not required but also not discretionary: fuel uplifted for tanker (the process of carrying fuel on a flight segment for the purposes of using it on subsequent flight segments, reflecting a business decision of the airline)
- Discretionary fuel: fuel uplifted for contingencies (fuel uplifted in the “contingency” category and fuel for non-required alternate airports).
As our dataset also includes values for SCF99 and SCF95 presented to the airline dispatcher at the time the fuel was uplifted, as well as the weather conditions allowing us to know if fuel for an alternate airport is required or not.
Using this dataset, we estimated the cost for all flights to carry 1. All fuel unused in flight and 2. Additional contingency fuel (excess fuel uplifted beyond a conservative fuel buffer) loaded onto a flight. The results of these calculations enable us to assess the potential of fuel loading reform to reduce the environmental impact of regulation. Clearly, the cost to carry all remaining fuel on board at the time of arrival represents a theoretical upper bound on the gains from eliminating excess fuel uplift. This upper limit could be attained only if the fuel required for a given flight could be precisely determined prior to departure. On the other hand, the cost to carry unnecessary contingency fuel – which we define as any contingency fuel above the SCF99 value plus fuel uplifted for non-required alternate airports – represents savings that are attainable without changes in the levels of uncertainty in today’s flight operations. It can therefore be considered a lower bound on the possible fuel savings from reducing discretionary fueling.
Let us present a few key findings before we estimate the broader cost to carry fuel on board. First, we find that virtually all flights land with the 45 minutes of required reserve fuel for holding; this fuel is basically considered a black box not to be used in flight. Regarding contingency fuel, we find that about 50% of the fuel at the time of arrival (meaning it is unused in flight) is not actually required to be on board due to federal regulations nor is it for the business decision of tanker. We also see that 75% of the flights have at least 13 minutes of additional contingency fuel (contingency fuel above SCF99, the reasonable buffer). In short, flights are departing with 13 minutes of fuel that is above a conservative contingency fuel buffer. Overall, we find that on a typical flight 4.48% of the fuel consumed is due to carrying fuel that is unused, while 1.04% of the fuel consumed is due to carrying additional contingency fuel above a reasonable buffer combined with loading fuel for unrequired alternates.
We also find that throughout the course of the year, our study airline burns 467 million lbs of fuel and emits 1.46 billion lbs of CO2 to carry all fuel it does not burn in flight. This airline burns 108 million lbs of fuel and emits 338 million lbs of CO2 to carry contingency fuel above a conservative buffer and fuel for unrequired alternates throughout the course of the year. The monetary costs of carrying this unused fuel are $223 million and $51.7 million, respectively, for our study year. If all airlines operating in the U.S. domestic aviation system fuel their aircraft in a similar manner to our study airline, the total fuel consumed due to carrying all fuel unused in flight for our study year would be 3.05 billion lbs resulting in 9.49 billion lbs of CO2; for additional contingency fuel and unrequired alternates these figures would be 707 million lbs of fuel and 2.20 billion lbs of CO2. Considering the total of transportation CO2 emissions, the contributions from airlines carrying extra fuel vary from .04% to .25%, depending on the metric considered. These percentages are small; however, in the context of broader transportation fuel consumption reduction goal it is well understood that CO2 reduction goals will be met with a suite of small reductions rather than single large-scale reductions.
To put the values of per-flight fuel consumed due to carrying fuel and the annualized fuel consumed, CO2 emitted, and cost incurred from carrying fuel into context, we compare these values to current aviation and transportation fuel-saving initiatives.
First we consider per-flight fuel savings initiatives. Consider the FAA estimates that precise navigation and continuous approach procedures at Seattle-Tacoma International Airport, implemented in 2014, will save Alaska Airlines 14 million lbs of jet fuel annually (about 130 lbs per flight or 60 kg per flight). The savings value from this specific, localized investment on a per-flight basis is very close to that from reducing additional contingency and unrequired alternate fuel (with a median value of 146 lbs per flight). Regarding ground-based initiatives, consider that there are numerous studies and federal initiatives focused on reducing fuel consumed by an aircraft during taxi out (from the gate to the runway); the findings from these studies show percentages of fuel savings that are directly in line with the savings from reducing contingency and unrequired alternate fuel uplift.
Regarding system-wide benefits, the FAA published estimates of the fuel savings benefits from their large-scale modernization initiative NextGen amounting to 38.7 billion gallons of fuel through 2030 or 2.4 billion gallons per year. If we assume all carriers fuel their domestic aircraft in a similar manner, our overall benefit pool for eliminating additional contingency and unrequired alternate fuel is 707 million lbs of fuel (and 2.20 billion lbs of CO2), or 29.5% of the entire annual fuel benefit from NextGen. Moreover, if it were possible to create a system in which aircraft could land with their fuel tanks containing only 45 min of reserve fuel and any required alternate reserve the benefit across the industry alone would be 1.50 billion gallons of fuel per year, 62.5% of the estimated value for NextGen. Given that the benefit pool from reducing additional contingency and unrequired alternate fuel is achievable (as it is fuel that is above a reasonable buffer), the implication is that it is possible to achieve 29.5% of the benefit of NextGen (which is estimated to cost $29 billion through 2030) by changing fuel loading practices rather than investing in technology and changing operational procedures of airports, airlines, and the airspace.
Consistent with U.S. Environmental Protection Agency recommendations that transportation emissions can be reduced by eliminating trips, we can convert our values to the equivalent number of flights that would have to be removed from the system to achieve the same savings from reducing fuel uplift. Considering that our airline operates about 720,000 flights per year and assuming all of these are on midsized aircraft, these values are the equivalent to cancelling all their domestic flights for 2.4 days (to be equal to the fuel burned from carrying additional contingency and unrequired alternate fuel) to 5.13 days (to be equal to the fuel burned from all fuel unused in flight) (see Figure 1).
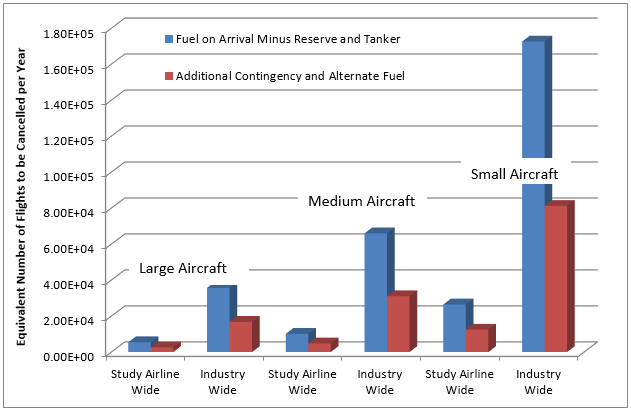
Finally, we compare the savings from reducing fuel uplift to the savings possible from reducing vehicle miles traveled on the highway system. Consider that a current (~20 mi/gal or 8.5 km/liter) vehicle emits about one pound of CO2 per vehicle-mile traveled (VMT) (or 0.28 kg of CO2 per vehicle-kilometer traveled). Using VMT figures from Chester and Horvath (2012), the savings from reducing the cost of carrying all fuel unused in flight from our study airline is the equivalent of removing 718 million VMT per year or the equivalent of removing 0.32% of the VMT in the state of California. If we consider eliminating additional contingency and unrequired alternate fuel from the study airline, the savings are 338 million VMT per year or the equivalent of removing 0.15% of the VMT in the state of California. If we generalize the equivalent VMT savings from reducing fuel uplift across the entire U.S. domestic aviation industry, we find that eliminating carrying all fuel on arrival from all U.S. domestic flights is equivalent to removing 4.68 billion VMT and 1.99% of California VMT, while reducing contingency and unrequired alternate fueling is equivalent to removing 2.20 billion VMT and 0.93% of California’s VMT.
The comparison of fuel burned due to contingency uplift to other savings showcase how simple changes in airline dispatcher behavior – resulting from airline or federal guidance encouraging dispatchers to add contingency fuel at the SCF99 level – could result in significant fuel savings. Reducing contingency fuel uplift to the SCF99 level and reducing and unrequired alternate fuel uplift would not require rule changes and would still maintain a large safety margin. While saving fuel from reducing uplift could come in the absence of federal guidance, federal intervention could help. Such reforms may either target rules that determine minimum fuel loads, behaviors that determine discretionary fuel loads above the minimum, or factors in the operating environment that shape behaviors or how the rules are applied. To start, the FAA could consider revising the role of federally-mandated reserve fuel. It is at the discretion of the airline if the reserve fuel is considered useable fuel for contingencies or if instead it is to be treated as protected and not to be used. Towards empowering airlines to reduce contingency fuel uplift beyond SCF99, the FAA could release guidance explicitly outlining how a portion of reserve fuel could be used for contingencies. Considering that fuel beyond SCF99 should (statistically) rarely be necessary, explicitly outlining how airlines could use the reserve fuel in these very unlikely situations could help reduce uplift.
Megan Ryerson
UPS Chair of Transportation, Weitzman SchoolMegan Ryerson is the UPS Chair of Transportation and Associate Chair of City and Regional Planning at the Stuart Weitzman School of Design.
Mark Hansen
Professor, University of California BerkeleyMark Hansen is a professor in the Department of Civil and Environmental Engineering at the University of California, Berkeley. He is also the co-director of the National Center of Excellence for Aviation Operations Research.
Lu Hao
Graduate Student, University of California BerkeleyLu Hao is a graduate student researcher in the Department of Environmental Engineering at the University of California, Berkeley.
Michael Seelhorst
Director of Operations, Revenue AnalyticsMichael Seelhorst is the director of operations research at Revenue Analytics, Inc.
Ayra, E.S., Insua, D.R., Cano, J., 2014. To Fuel or Not to Fuel? Is that the Question? Journal of the American Statistical Association 109, 465–476. doi:10.1080/01621459.2013.879060
Chester, M., Horvath, A., 2012. High-speed rail with emerging automobiles and aircraft can reduce environmental impacts in California’s future. Environ. Res. Lett. 7, 034012. doi:10.1088/1748-9326/7/3/034012
Chester, M.V., Horvath, A., 2009. Environmental assessment of passenger transportation should include infrastructure and supply chains. Environ. Res. Lett. 4, 024008. doi:10.1088/1748-9326/4/2/024008
Federal Aviation Administration, 2014. NextGen: The Business Case for the Next Generation Air Transportation System.
Karisch, S.E., Altus, S.S., Stojković, G., Stojković, M., 2012. Operations, in: Quantitative Problem Solving Methods in the Airline Industry, A Modeling Methodology Handbook Series: International Series in Operations Research & Management Science. Springer US.
Nikoleris, T., Gupta, G., Kistler, M., 2011. Detailed estimation of fuel consumption and emissions during aircraft taxi operations at Dallas/Fort Worth International Airport. Transportation Research Part D: Transport and Environment 16, 302–308. doi:10.1016/j.trd.2011.01.007
Lutsey, N., Sperling, D., 2009. Greenhouse gas mitigation supply curve for the United States for transport versus other sectors. Transportation Research Part D: Transport and Environment 14, 222–229. doi:10.1016/j.trd.2008.12.002
Ryerson, M.S., Hansen, M., Bonn, J., 2014. Time to burn: Flight delay, terminal efficiency, and fuel consumption in the National Airspace System. Transportation Research Part A: Policy and Practice 69, 286–298. doi:10.1016/j.tra.2014.08.024
Simaiakis, I., Khadilkar, H., Balakrishnan, H., Reynolds, T.G., Hansman, R.J., 2014. Demonstration of reduced airport congestion through pushback rate control. Transportation Research Part A: Policy and Practice 66, 251–267. doi:10.1016/j.tra.2014.05.014
Trujillo, A.C., 1996. Uncertainties that flight crews and dispatchers must consider when calculating the fuel needed for a flight (No. RTOP 505-64-53-01). NASA Langley Research Center; Hampton, VA United States.
Zou, B., Elke, M., Hansen, M., Kafle, N., 2014. Evaluating air carrier fuel efficiency in the U.S. airline industry. Transportation Research Part A: Policy and Practice 59, 306–330. doi:10.1016/j.tra.2013.12.003