Harvesting the Sun: On-Farm Opportunities and Challenges for Solar Development
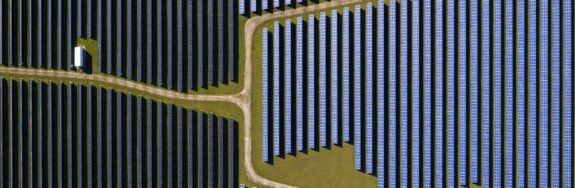
In the U.S., fossil fuel deposits are linked to land ownership, and many farmers have capitalized by selling their mineral rights to energy companies. Recent solar installations on farms are proving to be more profitable with fewer environmental impacts.
At a Glance
Key Challenge
If the U.S. wants to meet its ambitious targets for renewable energy development, the share of land directly affected by new renewable energy infrastructure would increase dramatically and may encroach on existing agricultural lands.
Policy Insight
On-farm solar development is increasingly becoming a financially viable and environmentally friendly alternative on American farmland. But realizing these co-benefits at scale will require a long-term commitment and innovative solutions from local, state, and federal policymakers.
Introduction
By 2040, the amount of land needed to meet the United States’ growing energy requirements will increase by 27%, directly affecting an estimated 200,000 square kilometers (sq. km.) of land with new energy development (Trainor et al. 2016, 1-16). This is the projected result of both a changing energy portfolio and increasing demand.
Over the last decade, for example, advances in drilling technology have unlocked considerable energy potential from 1.3 million sq. km. of land—roughly twice the size of Alaska—that had previously been ill-suited for conventional oil and gas development. Fossil fuel production demands constant land expansion as available resources are depleted. Production will continue to encroach on new land for as long as demand for these fuels persists.
If the U.S. sets ambitious targets for renewable energy development, with the ultimate goal of reaching net-zero carbon emissions from energy by 2050, the share of land directly affected by new renewable energy infrastructure would increase dramatically (Larson et al. 2020, 1-345). Just meeting existing electricity demand with photovoltaics would require approximately 290,000 sq. km. of land, assuming 150 MW/ sq. km. of electricity generation, a capacity factor of 25%, as well as perfect load balancing (U.S. Energy Information Administration, n.d.).1
In the lower 48 states, 63% of land is used for agricultural purposes (Economic Research Service, U. S. Department of Agriculture). As demand for energy infrastructure increases, land competition between energy and agricultural production will inevitably grow—as will the potential advantages of co-locating these land uses where possible.
We have already seen the shale boom drive mineral developers to many agricultural regions, and the result has been a surge in domestic fuel production and a significant secondary source of income for many farm operators (Hitaj and Suttles 2016, 1-47). In some states, like Oklahoma and Pennsylvania, oil and gas development leases provide up to 6% of gross cash farm income, and an even greater share of net income.
In 2014, more than 10% of farms in 9 American states received energy production-related payments. Average payments can be sizable, exceeding $150,000 in Pennsylvania and North Dakota. These leases can be immensely valuable to farm owners, since most American farms are small and depend on off-farm sources of income to remain operational (Economic Research Service, n.d; U. S. Department of Agriculture, n.d.).
Although the co-location of energy infrastructure on farmland has historically been mostly limited to oil and gas development, on-farm solar development is increasingly becoming a financially viable and environmentally friendly alternative on American farmland. On-farm solar development can help meet the country’s swelling demand for carbon-free energy, offer farmers and rural communities a consistent and long-term stream of income, and even boost agricultural productivity under the right circumstances.
However, realizing these co-benefits at scale will require a long-term commitment and innovative solutions from local, state, and federal policymakers. In this policy digest, we lay out why farmers choose to lease their mineral rights, the unpredictable costs of on-farm oil and gas development, and why solar could be a better alternative.
How Mineral Leasing Works
In the United States, most subsurface rights—the rights to minerals beneath the ground—are privately owned by individuals (Fitzgerald 2014, 1-7). Typically, energy companies interested in extracting minerals will lease land from owners rather than purchase the land outright. This is an effective way for the development companies to reduce capital expenses – reflecting their singular and short-term interest in the land.
The compensation structure for these land leases typically includes an upfront payment as well as a royalty, which reflects a share of the gross revenue of any oil or gas that is ultimately extracted (typically between 13 to 21%) (Brown et al. 2016, 23-38). Crucially, energy development companies can deduct expenses associated with transportation and processing from landowners’ royalty payments (Fitzgerald 2014, 1-7).
As one might expect, there is a substantial amount of information asymmetry between the lessee and the lessor in these arrangements. Company representatives have extensive experience negotiating agreements, while farm owners may only negotiate a mineral lease once in a lifetime. In addition, because oil and gas resources at a given site require expert analysis to approximate, the energy company generally has a more complete understanding of expected production than the mineral rights owner.
Fuel prices are also a considerable source of revenue variability. In 2020, for example, the U.S. government saw its royalties from mineral rights fall from $2 billion between March and June, compared to $4 billion in the same period last year, because of the precipitous drop in fuel prices and production induced by the COVID-19 pandemic (Knight 2020).
Landowners have little recourse in response to disruptions in expected revenue since contracts can last for many years. And when prices fall, the post-production costs deducted by developers can eat into farmers’ royalty checks, especially if their lease agreements do not address allowable deductions (Cusick and Sisk 2018).
Not only are oil and gas leases often uncertain value propositions, they also come with a number of serious economic and environmental risks for farmers (U.S. Geological Survey, n.d.). At best, development leads to increased traffic and noise pollution, and places increased demands on local water resources. At worst, oil and gas development leads to water and soil contamination and reduced land productivity (Environmental Protection Agency 2015, 1-25).
A typical shale gas well can use 2 to 4 million gallons of water during fracturing, the process by which gas resources are extricated from subterranean rock formations (U.S. Department of Energy 2009, 1-98). Wells can drive-up local water prices or compel farmers to modify their operations (Dutzik et al. 2012, 1-43).
Accidental water contamination or improper gas flaring can sicken or even kill livestock. Furthermore, according to a 2019 Energy Research & Social Science study that surveyed farmers in four midwestern states, many respondents reported relying on themselves or family to complete land reclamation efforts following oil or gas development (Haggerty et al. 2019, 84-92).
For farmers who own their mineral rights and are approached by a developer, the security of a secondary source of income—even one that comes with the uncertainties of energy land leases—can be attractive. The promise of additional revenue often outweighs the environmental risks, an indication of the substantial economic pressures many farmers face.
Yet for many rural communities, mineral leases may fail to provide much long-term benefit. The precise economic effect of natural gas development remains an area of active research. A 2016 study found that employment and wages can grow in the first four years of gas development, but decline to pre-boom levels over time (Komarek 2016, 1-17).
A 2014 study of the oil and gas boom in the American West in the 1970s and 1980s actually found that per capita incomes, following the bust, were 6% lower than pre-boom levels and that unemployment compensation remained elevated throughout the post-bust period (Jacobsen and Parker 2016, 1092-1128). The authors suggest that overspecialization in infrastructure and skills specific to the boom limited market participants’ ability to find new business and employment opportunities once the demand for extraction services receded and economic fundamentals changed.
Perhaps most importantly, a closer look at payment statistics reveals that the financial rewards of oil and gas development are not equally available to all American farmers, and instead largely accrue to a small subset (Hitaj et al. 2018, 1-31). In 2014, the top 10% of farmers receiving oil and gas payments received 18 times more money than the bottom 50% of farmers receiving payments. The mean payment to all farmers receiving oil and gas royalties was $43,736, dwarfing the median payment of just $6,600.
Is Solar a Better Option?
For all of their economic risks and environmental harms, mineral leases demonstrate an opportunity for the co-location of energy and agricultural production. On-farm solar (or agrivoltaics) can offer farmers and rural landowners a smaller environmental footprint and fewer economic risks than oil and gas development, while still providing a reliable secondary source of income. As the country’s energy demand affects more and more land, agrivoltaics can also play a crucial role in accelerating the transition to renewables.
First and foremost, solar panels present almost no risk of soil or water contamination when installed and maintained properly. In terms of water consumption, photovoltaic maintenance only requires enough water to occasionally wash dust and grime from panel surfaces (Clarke 2014). Compared to an oil or gas well, this water use is negligible.
Further, solar panels produce no additional toxic waste, and aside from soil disturbance during installation or removal, they have little long-term impact on the productivity of the land on which they are sited. While larger solar installations can have negative effects on soil and vegetation, there are a number of measures—like careful siting, prudent landscaping, and re-vegetation—that can mitigate these concerns (Dhar et al. 2020, 134602). In general, solar panels have a dramatically more favorable environmental profile than traditional sources of power generation (Turney and Fthenakis 2011, 3261-3270).
Solar power is also a flexible, reliable, and scalable source of energy, especially on agricultural land. Whereas oil and gas wells require a minimum of 5-10 acres of land, solar can be deployed to whatever scale a farm owner desires or is able to accommodate (MineralWise, n.d.). This means that solar can be developed on land that is already unused or unirrigated by farmers, minimizing disruptions to existing farm production.
In 2011, the National Renewable Energy Laboratory estimated that Colorado had over 1,200 sq. km. of non-irrigated corners of center-pivot irrigation fields (Roberts 2011, 1-11). This land could, in theory, support 890 sq. km. of solar fields without compromising agricultural productivity.
While a farmer’s opportunity to capitalize on mineral rights is entirely dependent on whether or not there is an accessible oil or gas basin, photovoltaics are an economically viable investment for landowners across the country, and solar power is at its most productive (Adeh et al. 2019, 11442) when installed on croplands (McDonnell 2020). While temperature and average cloud cover determine the capacity factor of cells, solar is already being successfully deployed from Arizona to Maine.
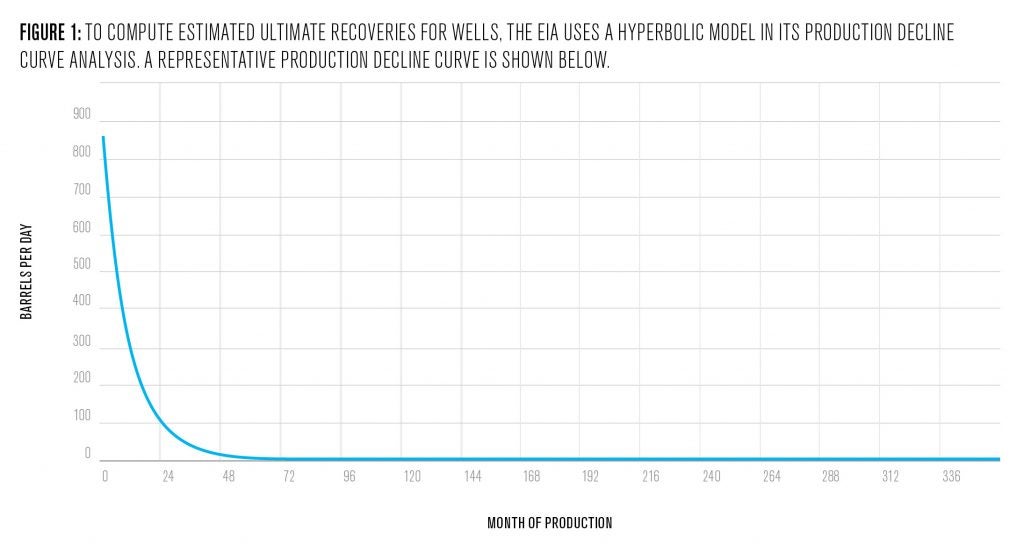
Solar power is also immune to hyperbolic declines in production, as is possible with oil and gas drilling (see Figure 1) (U.S. Energy Information Administration 2020). Instead, solar leases are long-term (Moore 2017), typically lasting around 20 years, with fixed rental contracts instead of royalties (White). This reduces the economic risk borne by landowners, and while there is certainly risk associated with long-term agreements, the fixed payment structure—as well as fairly predictable life-cycle costs—can help farmers avoid imbalanced negotiations with developers and plan for the future (Xiarchos and Vick 2011, 1-86).
In some cases, revenue from solar development can eclipse the revenue generated by harvest yields (Bookwalter 2019), though other studies have suggested that payback periods for on-farm solar projects are still too long (Colorado State University Extension, n.d., 45-48).
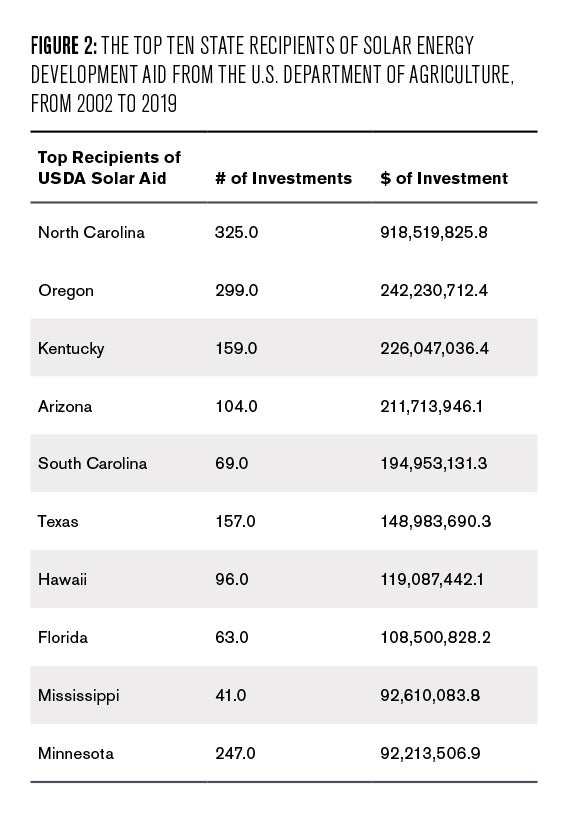
Still, the benefits of solar panels on farmland could extend far beyond simply providing a supplementary income source; they can, in the best case, actively enhance farm operations and improve agricultural yield. Agrivoltaics—the siting of elevated solar panels above crops, which continue to be cultivated—can confer a number of synergistic benefits, which oil and gas development cannot emulate (Barron-Gafford et al. 2019, 848-855).
Agrivoltaics are capable of reducing transpiration of water from plants and the evaporation of water from soil, thereby reducing farmers’ water use. Solar panels can also mitigate some of the light and heat stress that can have an adverse effect on crop photosynthesis.
Finally, transpired water has a cooling effect on solar panels, improving their efficiency by at least 1% (Tricoles 2017). While the effects of agrivoltaics on crop yield varies by species, some study results have shown a doubling in total fruit production and water efficiency in shade-tolerant and temperature-sensitive crops (Barron-Gafford et al. 2019, 848-855).
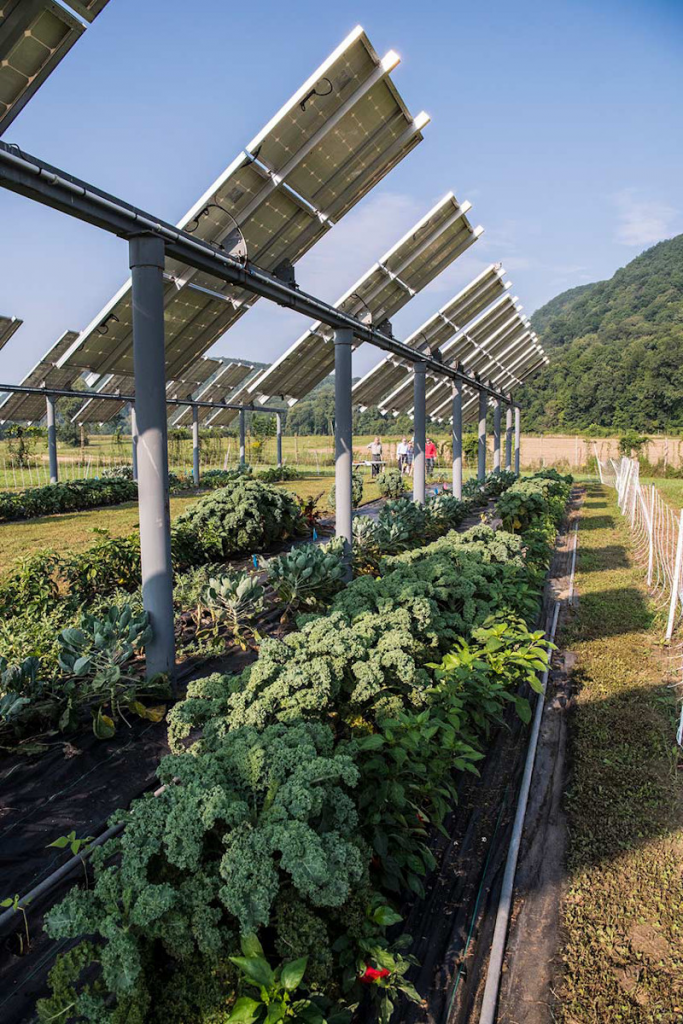
In the context of the wider economy, agrivoltaics can serve as a mitigant (Agostini et al. 2021, 116102) against market shocks or crop shortages and can help meet the energy demands of several farm operations such as pumping water, refrigeration, lighting, and sprinkler systems (Xiarchos and Vick 2011, 1-86). The benefits of agrivoltaics extend to livestock farming as well. The co-siting of photovoltaics on a rabbit farm, for example, was recently shown to reduce operating costs by up to 8%, increase revenue by 17%, and cut fencing-related costs (Lytle et al. 2021, 124476).
Remaining Challenges and Opportunities
The opportunities offered by on-farm solar development are considerable, especially when compared to mineral leases. However, there are some remaining economic and policy challenges that demand policy solutions before the full potential of co-locating agriculture and solar generation can be fully realized. These solutions would promote the (a) provision of public funds for rural energy development and incentive programs, (b) the circulation of tools and information that can help farmers make financially sound decisions, and (c) the implementation of streamlined land use policies to facilitate solar development and protect crop yields.
Fund Solar Projects
Continued public funding is necessary to encourage the adoption of solar resources and ensure that such projects make financial sense for farm operators. There are already a number of (Tennessee Department of Energy and Conservation 2020; Massachusetts Farm Energy Program, n.d.) state and federal funding opportunities for farmers interested in investing in on-farm renewable energy projects, including a 30% federal business energy investment tax credit and a 25% Rural Energy for America Program grant from the U.S. Department of Agriculture (neither of which are available to oil and gas developers) (Hay 2016, 1-27).
However, for agricultural land to host meaningful solar generation capacity and support a rapid transition to renewables, these funding opportunities ought to be accessible to a much wider community of farmers. Specifically, federal agencies, like the USDA, should direct greater public funding toward on-farm solar deployment. The availability of external funding is a significant determinant of the ultimate profitability and size of renewable energy systems adopted by farmers (Bazen and Brown 2009, 748-754; Beckman and Xiarchos 2013, 322-330).
In recent years, the federal government has aggressively stepped up its support of solar projects in rural America. Between 2002 and 2019, the USDA distributed over $7.7 billion in grant aid to support renewable energy development in rural communities (USDA, n.d.). Along with anaerobic digesters, solar projects have been the largest recipients of this USDA support in the past five years.
These targeted grants and loan guarantees have helped small businesses cut their energy costs and energy consumption (USDA 2019). In 2015 alone, solar projects financed by the USDA’s Rural Energy for America Program generated 530,000 MWhs of electricity (Hitaj and Suttles 2016, 1-47). Still, federal support for investment in agricultural infrastructure remains relatively modest and should be significantly expanded in order to meet changing energy demands.
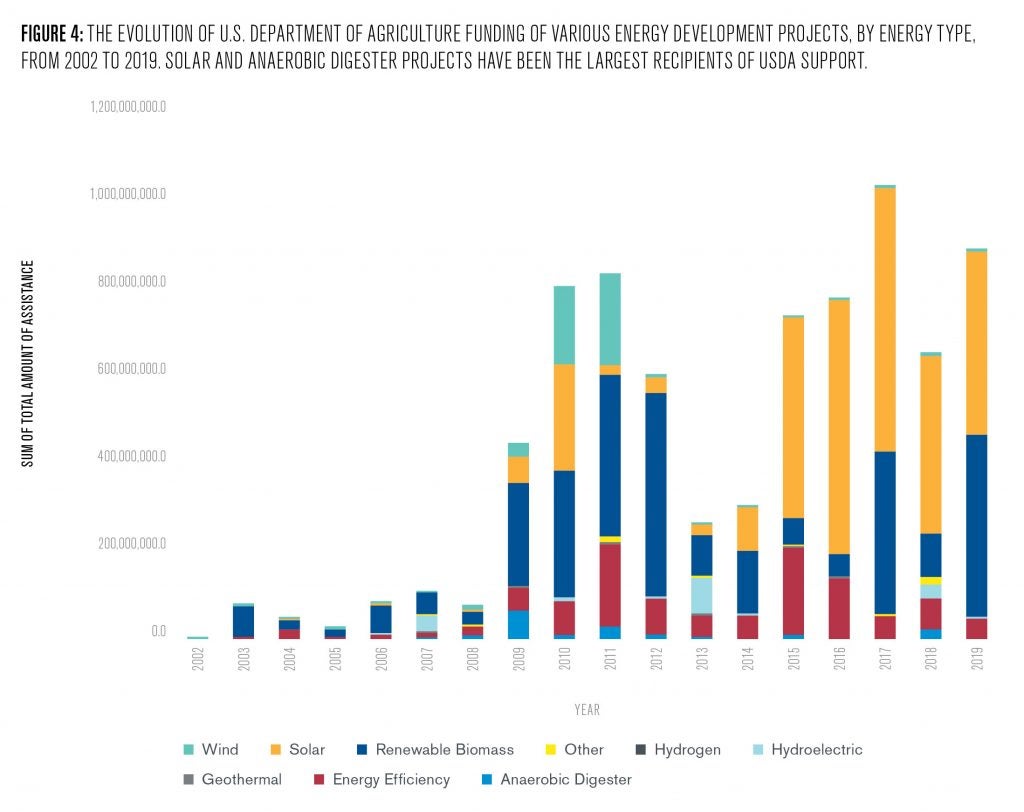
Federal loan and grant programs still play a critical role in making solar development a profitable proposition for farm operators and in sustaining investor interest (Petrovich et al. 2021, 106856). In 2015 and 2016, Colorado State University conducted 30 solar assessments for farmers interested in renewable systems deployment in pivots—land left unused owing to center-pivot irrigation (Colorado State University Extension, n.d. 45-48). Those researchers found that the average solar array would have generated lifetime energy savings of $156,000, in addition to $23,000 in payments for excess electricity sold back to the grid. The up-front cost of the average solar array was $137,000, before incorporating any federal grants and tax credits. Accounting for such credits, the total cost would fall to $71,000, significantly reducing the payback period and resulting in a return on investment of 4.7%.
Inform Farmers
Farm operators and rural communities need to be empowered with the information to make financially and environmentally sound decisions regarding on-farm energy development. One of the central goals of policymakers interested in facilitating on-farm solar development should be to help clarify the full financial picture of a proposed project. Absent such support, it would be easy to discount on-farm renewable energy based on revenue figures alone: According to a USDA. analysis, in 2014, the average payment to farm operators for leasing wind rights was $8,287, substantially less than the average payment of $43,736 from oil and gas (Hitaj et al. 2018, 1-31). While the USDA did not consider revenues associated with on-farm solar projects in that study, modern solar and wind installations have similar costs/kW and capacity factors in the same ballpark, so landowners can expect lease revenue from solar projects to be similarly modest (Mey 2020; SolarLandLease, n.d.). It is worth noting, however, that wind power is significantly less energy dense than photovoltaic power in terms of kW/acre. This means that while costs/kW and capacity factors may be similar across both technologies, photovoltaics may offer farmers and developers the flexibility to generate more electricity from the same acreage.
Sound information and technical guidance, however, could allow on-farm solar projects to be financially viable investments that circumvent many of the risks associated with traditional oil and gas development. A key advantage of solar development, over oil or gas, is that solar radiation is much easier to estimate than subsurface mineral availability.
Whereas oil and gas are found in relatively dense pockets of geological formations and require extensive site exploration to uncover and approximate, solar radiation is easily mappable based on geographic location, local topography, and surrounding vegetation. In fact, the National Renewable Energy Laboratory offers a solar calculator tool online that allows users to estimate the performance of solar facilities, based on their location and other variables (National Renewable Energy Laboratory, n.d.).
But solar radiation is just one of several inputs. For farmers considering leasing their land for solar development, the value of their land and the range of possible per-acre rental fees they could collect is essential information needed for negotiations with developers. According to Strategic Solar Group, annual per-acre rents for larger tracts of solar land can range from $300 to $800 depending on a state’s average capacity factor and land availability (White).
To help farmers navigate these financial considerations—for land leases and personal projects alike—federally-supported, no-cost energy audits should be made available to all farm owners (New York State Energy Research and Development Authority, n.d.). These audits would help operators identify possible applications of solar power and understand the costs, savings, and payback periods of possible solar development (among other energy-saving and emissions-reducing measures).
Between 2016 and 2019, CSU Rural Energy Center administered its Farm Assessments for Solar Energy program, which provided 60 free evaluations to farmers about the feasibility of solar installations on their properties (Colorado State University Extension, n.d.). Colorado’s Energy Office also administers an Agricultural Energy Efficiency program, which provides free audits for eligible farms seeking to reduce energy expenses and implement cost-saving measures (Colorado Energy Office, n.d.).
Further expanding the reach of such programs—and leveraging emerging technologies such as LiDAR to improve and streamline auditing—could protect rural landowners in negotiations in a way that has never really been possible with oil and gas leases. These audits could give landowners confidence in moving forward with rental agreements or personal development projects—lending assurance that their investments are sound and ultimate revenues are fair, even if those revenues are relatively modest.
Clarify Policies
Land use, planning, and energy policies need to be clarified and made more consistent. On-farm solar development has the potential to directly compete with existing cropland if not planned and developed with sustained or improved agricultural productivity in mind. Finding this balance remains a major focus for state and local officials and policymakers (Bergan and Braun 2019).
The Grow Solar Initiative, a USDA-funded effort to boost the solar production potential of three Midwestern states, observes that regulatory and statutory inconsistencies for siting projects can be major obstacles to the growth of the solar industry (Grow Solar 2015). As the opportunities for shared land use become better understood, local and state governments need to outline clear and detailed guidelines for what constitutes appropriate and allowable shared use of agricultural land.
In 2019, for example, North Dakota’s Public Service Commission approved the construction of a 200 MW utility-scale project on 1,600 acres of prime farmland (Lee 2019). Under existing North Dakota laws, this land would have been excluded from development if the overall effect on agricultural yields was perceived to be too large. Existing laws, however, did not specifically prescribe what constituted a large effect on agricultural yields, so the commission had to deliberate (unearthing microform documents from the 1970s in the process) before reaching a decision.
Like North Dakota, Michigan has had to wrestle with decades-old laws blocking more rapid solar development. The state’s Farmland and Open Space Preservation Program, passed in 1975, requires participating farmers to maintain their farmland for agricultural uses in exchange for tax incentives and exemptions (Michigan Department of Agriculture and Rural Development). But the state decided in 2017 that commercial solar development was not a permissible activity on land preserved by the program, excluding one-third of the state’s farmland from solar electricity generation (the program covered 3 million acres in 2020) (Malewitz 2019; Michigan Department of Agriculture & Rural Development 2020, 1-20).
Farmers interested in solar development could have exited the program, but they would have had to pay back the previous seven years of tax credits along with 6% interest—a prohibitive barrier (Malewitz 2019). It took another two years before the state revised its policy, allowing solar development for commercial and personal purposes on preserved farmland (Michigan Department of Agriculture & Rural Development 2019).
Final Thoughts
The use of agricultural land for solar electricity generation can support the U.S. farm sector, strengthen rural economies, and facilitate the country’s energy transition. The shale gas revolution of the last decade has offered valuable lessons for farmers and energy developers about how energy lease agreements should be structured in order to both promote energy development and protect farmers, local resources, and surrounding ecosystems. On-farm solar power eliminates many of the most serious environmental risks of oil and gas development and can, if deployed correctly, increase the productivity of crops and livestock.
However, inconsistent regional land use policies, insufficient federal funding for development and research, and the inadequate availability of information mean that the full potential of solar development on American farmland has yet to be realized. The abundance of agricultural land in the United States could be a competitive advantage in national efforts to decarbonize, but until the necessary policy tools are leveraged, it is more likely to create unnecessary land competition.
Anuj Krishnamurthy
Global Food Systems InitiativeAnuj Krishnamurthy is a research assistant for the Global Food Systems Initiative. He is currently a student in Penn’s master of computer and information technology program.
Oscar Serpell
Deputy DirectorOscar Serpell oversees all student programming, alumni engagement, faculty and student grants, and visiting scholars. He is also a researcher, writer, and policy analyst working on research initiatives with students and Center partners.
Adeh, Elnaz H., Stephen P. Good, M. Calaf, and Chad W. Higgins. 2019. “Solar PV Power Potential is Greatest Over Croplands.” Scientific Reports 9, no. 1. Accessed April 22, 2021: https://doi.org/10.1038/s41598-019-47803-3.
Agostini, A., M. Colauzzi, and S. Amaducci. 2021. “Innovative Agrivoltaic Systems to Produce Sustainable Energy: An Economic and Environmental Assessment.” Applied Energy 281. Accessed April 22, 2021: https://www.sciencedirect.com/science/article/pii/S0306261920315245
Barron-Gafford, Greg, Mitchell Pavao-Zuckerman, Rebecca L. Minor, Leland F. Sutter, Isaiah Barnett-Moreno, Daniel T. Blackett, Moses Thompson, et al. 2019. “Agrivoltaics Provide Mutual Benefits Across the Food–Energy–Water Nexus in Drylands.” Nature Sustainability 2, no. 9 848-855. Accessed April 22, 2021:https://doi.org/10.1038/s41893-019-0364-5.
Bazen, Ernest F. and Matthew A. Brown. 2009. “Feasibility of Solar Technology (Photovoltaic) Adoption: A Case Study on Tennessee’s Poultry Industry.” Renewable Energy 34, no. 3 748-754. Accessed April 22, 2021: https://www.sciencedirect.com/science/article/pii/S0960148108001742.
Beckman, Jayson and Irene M. Xiarchos. 2013. “Why Are Californian Farmers Adopting More (and Larger) Renewable Energy Operations?” Renewable Energy 55, 322-330. Accessed April 22, 2021: https://www.sciencedirect.com/science/article/pii/S0960148112007094.
Bergan, Sara and Thomas Braun. 2019. “Balancing Solar Development and Preservation of Agricultural Lands in the Midwest.” PV Magazine. Accessed April 22, 2021: https://pv-magazine-usa.com/2019/08/27/balancing-solar-development-and-preservation-of-agricultural-lands-in-the-midwest/
Bookwalter, Genevieve. 2019. “The Next Money Crop for Farmers: Solar Panels.” The Washington Post. Accessed April 22, 2021: https://www.washingtonpost.com/business/economy/the-next-money-crop-for-farmers-solar-panels/2019/02/22/2cf99e8c-3601-11e9-854a-7a14d7fec96a_story.html
Brown, Jason P., Timothy Fitzgerald, and Jeremy G. Weber. 2016. “Capturing Rents from Natural Resource Abundance: Private Royalties from U.S. Onshore Oil & Gas Production.” Resource and Energy Economics 46, (2016): 23-38. Accessed April 22, 2021: https://www.sciencedirect.com/science/article/pii/S0928765516300355.
Clarke, Chris. 2014. “Fact Check: How Much Water Does Solar Power Really Use?” KCET. Accessed April 22, 2021: https://www.kcet.org/redefine/fact-check-how-much-water-does-solar-power-really-use
Colorado Energy Office. n.d. “Agricultural Energy Efficiency.” Accessed April 22, 2021: https://energyoffice.colorado.gov/clean-energy-programs/agricultural-energy-efficiency
Colorado State University Extension. n.d. “Farm Assessments for Solar Energy (FASE).” Accessed April 22, 2021: http://rec.colostate.edu/fase/
Cusick, Marie and Amy Sisk. 2018. “Millions Own Gas and Oil Under their Land. Here’s Why Only Some Strike It Rich.” National Public Radio. Accessed April 22, 2021: https://www.npr.org/2018/03/15/592890524/millions-own-gas-and-oil-under-their-land-heres-why-only-some-strike-it-rich
Dhar, Amalesh, M. A. Naeth, P. D. Jennings, and Mohamed Gamal El-Din. 2020 “Perspectives on Environmental Impacts and a Land Reclamation Strategy for Solar and Wind Energy Systems.” Science of the Total Environment 718. Accessed April 22, 2021: https://www.sciencedirect.com/science/article/pii/S0048969719345930.
Dutzik, Tony, Elizabeth Ridlington, and John Rumpler. 2012. “The Costs of Fracking: The Price Tag of Dirty Drilling’s Environmental Damage.” Environment Maryland Research & Policy Center. Accessed April 22, 2021: https://www.slideshare.net/MarcellusDN/the-cost-of-fracking-environment-maryland-documents-the-dollars-drained-by-dirty-drilling
Environmental Protection Agency. 2016. “U.S. EPA. Hydraulic Fracturing for Oil and Gas: Impacts from the Hydraulic Fracturing Water Cycle on Drinking Water Resources in the United States (Final Report).” Accessed April 22, 2021: https://cfpub.epa.gov/ncea/hfstudy/recordisplay.cfm?deid=332990
Freeman, Weldon & Jay Fletcher. 2019. “USDA Invests in Energy Efficiency Improvements to Help Farmers, Rural Businesses and Ag Producers Lower Energy Costs.” U.S. Department of Agriculture. Accessed May 6, 2021: https://www.usda.gov/media/press-releases/2019/08/19/usda-invests-energy-efficiency-improvements-help-farmers-rural
Grow Solar. 2015.“Three State Regional Analysis: Creating ‘Solar-Ready’ Communities.” Midwest Renewable Energy Association. Accessed May 6, 2021: https://www.growsolar.org/wp-content/uploads/2015/05/Three-State-Regional-Analysis.pdf
Haggerty, Julia H., Kristin K. Smith, Jason Weigle, Timothy W. Kelsey, Kathryn Bills Walsh, Roger Coupal, David Kay, and Paul Lachapelle. 2019. “Tradeoffs, Balancing, and Adaptation in the Agriculture-Oil and Gas Nexus: Insights from Farmers and Ranchers in the United States.” Energy Research & Social Science 47, 84-92. Accessed May 6, 2021: https://www.sciencedirect.com/science/article/pii/S2214629618309071.
Hay, F. John. 2016. “Solar Electric Investment Analysis.” University of Nebraska-Lincoln, Accessed May 6, 2021: https://extensionpublications.unl.edu/assets/pdf/ec3008.pdf
Hitaj, Claudia, Jeremy Weber, and Ken Erickson. 2018. “Ownership of Oil and Gas Rights: Implications for U.S. Farm Income and Wealth.” U.S. Department of Agriculture, Accessed May 6, 2021: https://www.ers.usda.gov/webdocs/publications/89325/eib-193.pdf?v=1381.1
Jacobsen, Grant D. and Dominic P. Parker. 2016. “The Economic Aftermath of Resource Booms: Evidence from Boomtowns in the American West.” The Economic Journal 126, no. 593: 1092-1128, Accessed May 6, 2021: https://doi.org/10.1111/ecoj.12173.
Knight, Chris. 2020. “US Energy Royalties Drop $2bn during Pandemic.” Argus Media. Accessed May 6, 2021: https://www.argusmedia.com/en/news/2124781-us-energy-royalties-drop-2bn-during-pandemic
Komarek, Timothy M. 2016. “Labor Market Dynamics and the Unconventional Natural Gas Boom: Evidence from the Marcellus Region.” Resource and Energy Economics 45: 1-17, Accessed May 6, 2021: https://www.sciencedirect.com/science/article/pii/S092876551630077X.
Lee, Demetria. 2019. “North Dakota Considers Changes to Farmland Rule to Allow Solar Projects.” Energy News Network Accessed May 6, 2021: https://energynews.us/2019/04/12/north-dakota-considers-changes-to-farmland-rule-to-allow-solar-projects/
Lustgarten, Abraham. 2013 “Unfair Share: How Oil and Gas Drillers Avoid Paying Royalties.” ProPublica. Accessed May 6, 2021: https://www.propublica.org/article/unfair-share-how-oil-and-gas-drillers-avoid-paying-royalties
Lytle, William, Theresa K. Meyer, Nagendra G. Tanikella, Laurie Burnham, Julie Engel, Chelsea Schelly, and Joshua M. Pearce. 2021. “Conceptual Design and Rationale for a New Agrivoltaics Concept: Pasture-Raised Rabbits and Solar Farming.” Journal of Cleaner Production 282. Accessed May 6, 2021: https://www.sciencedirect.com/science/article/pii/S0959652620345200.
Malewitz, Jim. 2019.”Gov. Gretchen Whitmer Opens Up More Michigan Farmland to Solar Power.” Bridge Michigan. Accessed May 6, 2021: https://www.bridgemi.com/michigan-environment-watch/gov-gretchen-whitmer-opens-more-michigan-farmland-solar-power
Massachusetts Farm Energy Program. n.d. “Funding Resources: Energy Efficiency Projects.” Massachusetts Department of Agricultural Resources. Accessed May 6, 2021: https://massfarmenergy.com/get-funding/
McDonnell, Tim. 2020. “Can Farmland Fix Solar Power’s Real Estate Problem? ” Quartz. Accessed May 6, 2021: https://qz.com/1913868/why-agricultural-land-is-better-than-rooftops-for-solar-panels/
Mey, Alex. 2020 “Average U.S. Construction Costs for Solar and Wind Generation Continue to Fall.” U.S. Energy Information Administration. Accessed May 6, 2021: https://www.eia.gov/todayinenergy/detail.php?id=45136
Michigan Department of Agriculture & Rural Development. 2020. “2020 Farmland Preservation Annual Report.” Accessed May 6, 2021: https://www.michigan.gov/documents/mdard/2020_Farmland_Preservation_Annual_Report_717862_7.pdf
Michigan Department of Agriculture and Rural Development. n.d. “The Farmland and Open Space Preservation Program.” Accessed May 6, 2021: https://www.michigan.gov/mdard/0,4610,7-125-1599_2558-10301–,00.html
Michigan Department of Agriculture and Rural Development. 2019. “Gov. Whitmer, MDARD to Allow Commercial Solar Panels on Michigan Farmland.” Accessed May 6, 2021: https://www.michigan.gov/mdard/0,4610,7-125–498799–,00.html
MineralWise. n.d. “What is Fracking?” Accessed May 6, 2021: https://mineralwise.com/what-is-fracking/
Moore, Robert. 2017. “Weigh Risks before Signing Solar Lease.” Farm Progress. Accessed May 6, 2021: https://www.farmprogress.com/land-management/weigh-risks-signing-solar-lease
National Renewable Energy Laboratory. n.d. “PVWatts Calculator.” Accessed May 6, 2021: https://pvwatts.nrel.gov/
New York State Energy Research and Development Authority. n.d. “Agriculture Energy Audit Program.” Accessed May 6, 2021: https://www.nyserda.ny.gov/all-programs/programs/agriculture-energy-audit
Office of Fossil Energy. 2009. “Modern Shale Gas Development in the United States: A Primer” U.S. Department of Energy. Accessed May 6, 2021: https://www.energy.gov/fe/downloads/modern-shale-gas-development-united-states-primer
Petrovich, Beatrice, Stefano Carattini, and Rolf Wüstenhagen. 2021. “The Price of Risk in Residential Solar Investments.” Ecological Economics 180 Accessed May 6, 2021: https://www.sciencedirect.com/science/article/pii/S0921800919321780.
Roberts, Billy. 2011.“Potential for Photovoltaic Solar Installation in Non-Irrigated Corners of Center Pivot Irrigation Fields in the State of Colorado.” National Renewable Energy Laboratory. Accessed May 6, 2021: https://www.nrel.gov/docs/fy11osti/51330.pdf
SolarLandLease. n.d. “Lease Rates for Solar Farms: How Valuable is My Land?” Accessed May 6, 2021: https://www.solarlandlease.com/lease-rates-for-solar-farms-how-valuable-is-my-land
Tennessee Department of Energy and Conservation. 2020. “Clean Tennessee Energy Grants.” Accessed May 6, 2021: https://www.tn.gov/environment/program-areas/opsp-policy-and-sustainable-practices/business-and-private-sector/clean-tennessee-energy-grants.html
Tricoles, Robin. 2017. “UA Researchers Plant Seeds to Make Renewable Energy More Efficient” The University of Arizona. Accessed May 6, 2021: https://news.arizona.edu/story/ua-researchers-plant-seeds-make-renewable-energy-more-efficient?utm_source=uanow&utm_medium=email&utm_campaign=
Turney, Damon and Vasilis Fthenakis. 2011. “Environmental Impacts from the Installation and Operation of Large-Scale Solar Power Plants.” Renewable and Sustainable Energy Reviews 15, no. 6: 3261-3270, Accessed May 6, 2021: https://www.sciencedirect.com/science/article/pii/S1364032111001675
U.S. Department of Agriculture. n.d. “Energy Investments Map.” Accessed May 6, 2021: https://www.usda.gov/energy/maps/maps/Investment.htm
U.S. Energy Information Administration. 2020. “Production Decline Curve Analysis in the Annual Energy Outlook 2020.” Accessed May 6, 2021: https://www.eia.gov/analysis/drilling/curve_analysis/
U.S. Geological Survey. n.d. “What Environmental Issues Are Associated with Hydraulic Fracturing?” Accessed May 6, 2021: https://www.usgs.gov/faqs/what-environmental-issues-are-associated-hydraulic-fracturing?qt-news_science_products=0#qt-news_science_products
Weiner, Cary and Brent Young. n.d. “Solar and Wind Assessments for Pivots” Rural Connections 45. Accessed May 6, 2021:http://ruralenergy.colostate.edu/wp-content/uploads/2020/04/SWAP-article-1.pdf
White, Michael. n.d. “What is the Average Solar Farm Lease Rate?” Accessed May 6, 2021: https://strategicsolargroup.com/what-is-the-average-solar-farm-lease-rate/
Xiarchos, Irene M. and Brian Vick. 2011. “Solar Energy Use in U.S. Agriculture: Overview and Policy Issues.” U.S. Department of Agriculture. Accessed May 6, 2021: https://naldc.nal.usda.gov/download/49148/PDF
- Author’s calculation: sq. km. = (((annual electricity demand in MWh/365 days)/24hours)*4)/150 MW [↩]