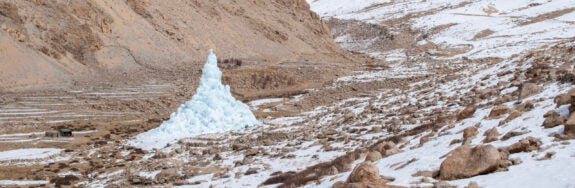
In vast arid and semi-arid regions of the world, the meltwater originating from the cryosphere represents a life-sustaining water source. One adaptive strategy, transferred from the Indian Himalayas to the Chilean Andes, may help alleviate glacier loss and drought.
At A Glance
Key Challenge
In Chile, glacier rights are complicated by the fact that water is privatized. Glaciers may be located within private property or intervened by mining operations. Projects depending on glacier meltwater must address impacts of the country’s existing legal framework and political economic model.
Policy Insight
It is important to take seriously the socioeconomic and historical dynamics of human–glacier–water interactions. Before engineering solutions, we must probe the structural issues that influence water access, glacier protection, and high mountain ecosystem management.
This paper is published in collaboration with The Water Center at Penn. Special thanks to academic reviewer Tony Sauder, senior advisor at The Water Center.
Introduction
Anthropogenic induced climate change is making itself felt and known through elemental state-change. The loss, disappearance, thawing, and retreat of ice and alterations in the broader cryosphere (including snow, glaciers, permafrost, lake and river ice) have become salient indicators in scientific and community assessments of climate change. Snow cover, glaciers, and permafrost are projected to continue to decline in almost all regions of the world throughout the 21st century (IPC 2019).
It is widely recognized that as ice disappears, sea levels rise, albeit with uneven geographical and socio-ecological consequences. Glacier retreat and instability carries with it risks of devastating meltwater release, which can in turn flood downstream communities, where vulnerabilities will closely mirror indices of inequality (Carey 2010).
Furthermore, in vast arid and semi-arid regions, such as Ladakh in northwestern India, and South American countries, such as central Chile, Peru, Bolivia, and Argentina, the meltwater originating from different components of the Himalayan and Andean cryospheres represent a crucial water source, sustaining human consumption, agriculture, mountain ecosystems, tourism, and hydro-electric generation, as well as holding significant spiritual and everyday importance for the life-worlds of diverse Indigenous peoples, mountain, and foothill communities (Krupnik et al. 2010; Cruickshank 2005).
Interest in glacier grafting or what local communities in the Himalayan region call “glacier mating” and other “ice cultivation” practices can be traced back centuries among the upper Indus Basin in Ladakh (Gagné 2016; Nüsser and Baghel 2016) and various locations in northern Pakistan (Schmidt and Nüsser 2017).
Techno-scientific oriented glacier preservation started in the European Alps in the 1960s, although most experimentation has taken place after 2000, when attention to ice and the melting cryosphere has become a pressing matter of global concern. Ice blankets in Europe, the creation of “artificial glaciers,” and ice stupas designed to refreeze glacier meltwater and facilitate water storage, are examples of relatively recent techno-scientific interventions into a melting and thawing world (Dodds and Sörlin 2022).
Low-cost engineering interventions relying primarily on temperature and gravity, such as “artificial glaciers” and frozen “water towers” or ice stupas, have gained increased international attention. They can be constructed at much lower altitudes than naturally occurring glaciers and are purported to be capable of bridging the critical gap in water availability by providing reservoirs that melt earlier in agricultural seasons.
These projects, originally built in the Indian Himalayas, are touted as an effective way to provide solutions to water shortage in cold arid regions and in areas where decreased precipitation and increased temperatures coincide with stream flow reduction and glacier retreat.
Since 2010, the arid and semiarid regions of the northern and central Chilean Andes have experienced what is being called a “megadrought” (Garreaud et al. 2020). This situation is exacerbated by the country’s privatized water system (Tamayo Grez and Carmona López 2019).
South American scientists have demonstrated that the agricultural productivity and freshwater provision for around 12 million people in the center region of Chile is strongly linked to the meltwater from the cordillera’s cryoforms (Crespo et al. 2020; Pena and Nazarala 1987). In response to the conditions produced by the ongoing drought and the effects of climate change, a group of Chilean engineers inspired by the Ladakhi ice stupas have begun a pilot project to build similar structures in Chile’s central Andes.
In this report, I explore how ice stupas functionally mimic glacier meltwater flow, with little resemblance to the complex ecosystems of glaciers. I discuss the potential of Global South-to-South transfer of technical interventions from the Indian Himalayas to the Chilean Andes. I also provide considerations about the transfer of engineer-based climate change adaptive strategies in response to glacier loss and drought.
Situation Analysis in Chile
The Andes Cordillera contains the most diverse cryosphere on Earth, including extensive areas covered by seasonal snow, numerous tropical and extratropical glaciers, and many mountain permafrost landforms (Masiokas et al. 2020). According to the 2022 national glacier inventory, Chile is home to 26,169 glaciers and roughly 80% of the glaciers in South America (DGA 2022).
Chile’s unique geography provides an extraordinary variety of climatic conditions and availability of water resources; however, most of its population is located in arid and semiarid climates where water availability is limited and prone to fluctuations based on reduction in precipitation and precipitation switches from snow to rain. Both phenomena alter the buffering effect of glacier meltwater in summer seasons and prolonged dry periods (Valdés-Pineda et al. 2014).
Due to the narrow distance between the Chilean Andes and Pacific Ocean, many of the country’s rivers are short, steeply graded, and torrential. This phenomenon has led to a historic discourse and institutional imperative, influencing energy policy, to prevent water from being “wasted into the sea” (Hernando-Arrese and Tironi 2019). In addition, under the military dictatorship of Pinochet (1973-1990), a Water Code was introduced that allowed private property rights over water (water use rights for consumption and non-consumption, for example for generating electricity). This created what is widely recognized to be the most aggressive neoliberal water regime in the world that further deepened in 1998, following the democratic transition when sanitation was also privatized. (Budds 2009).
According to the Chilean Defense Movement for Access to Water, Land and Environmental Protection (MODATIMA), in 2020, 77% of the consumption use rights were held by the agricultural and forestry sector, 13% by the mining sector, 7% by the industrial sector, and approximately 3% by the health sector. As for the rights for the use of water that is not consumed, 81% were in the hands of an Italian public-private company (CIVICUS 2020). The owners of water use rights can sell, or lease water use in the marketplace.
After eleven years of congressional discussion, the Chilean Water Code was reformed in January 2022. Changes were made to the premise of water rights by guaranteeing water for human consumption, not only for drinking but also for sanitation and daily subsistence.
The new law establishes that shares of water will be granted under a 30-year renewable concession. The volume of water allocated per share is to be based on the availability of the supply source, or sustainability of the aquifer in the case of underground water. Authorities may grant water use shares for shorter periods, if necessary, and may also decline to renew them (USDA 2022). It is yet to be seen how effective these reforms will be in guaranteeing the human right to water and sanitation, as well as greater water conservation.
The nexus between ongoing water conflicts and long-term climate projections do not present an encouraging picture for this region of the Andes, as they indicate a warming trend and a greater recurrence of droughts in the coming decades (CR2 2015). Subsequently, the Zero Celsius Degree Isotherm or freezing level within the Andes Mountain range will continue to rise, resulting in sustained glacier retreat (Dussaillant et al. 2019).
What is an Ice Stupa?
In 2020, a group of Santiago-based engineers saw a video of ice stupa projects in Ladakh, India. This conical form of ice reservoir was first designed in 2013 by mechanical engineer, educator, and active public figure, Sonam Wangchuk, and his team from the NGO, Students’ Educational and Cultural Movement of Ladakh (SECMOL). They began work on the project in response to climate-induced drought conditions and increasingly unreliable irrigation reserves for Himalayan farmers living downstream.
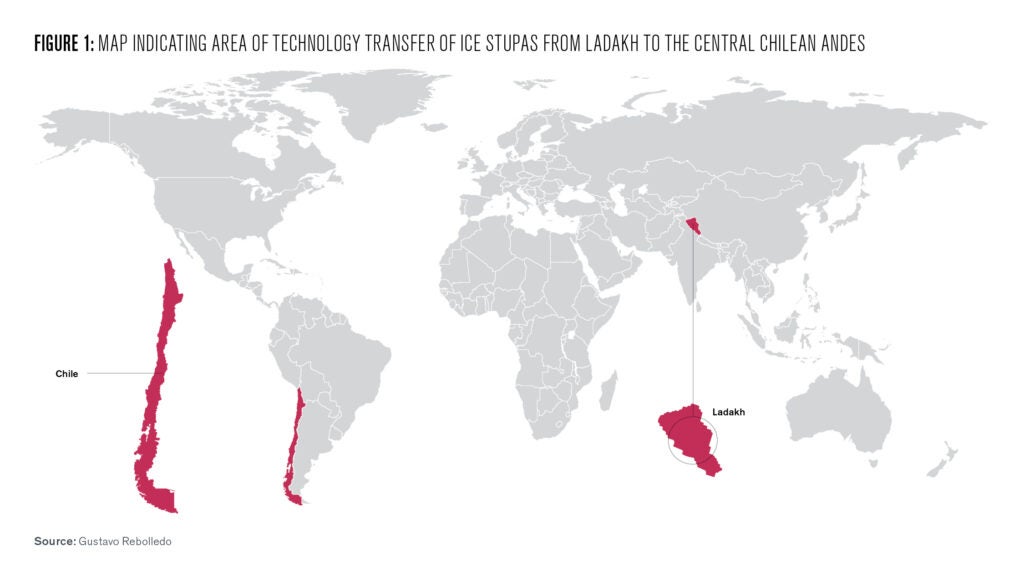
Ice stupas work by funneling water from a high-mountain stream through a pipe raised well above the ground. The energy source is the movement of water, a combination of gravity and pressure. As water exits the pipe at the stupa site, it is projected into the cold air through a fountainlike sprinkler, freezing almost immediately on top of a structure constructed below (See Photo 1).

The result is a mounded stupa-shaped block of ice that can last as a frozen mass well after surrounding snow has melted, thereby storing water that would have been unusable for agriculture during the winter for use in the spring and summer months (Clause 2016). The shape of these ice mounds resembles the region’s ubiquitous Buddhist masonry stupas; hence their name: ice stupas (See Photos 2 and 3).
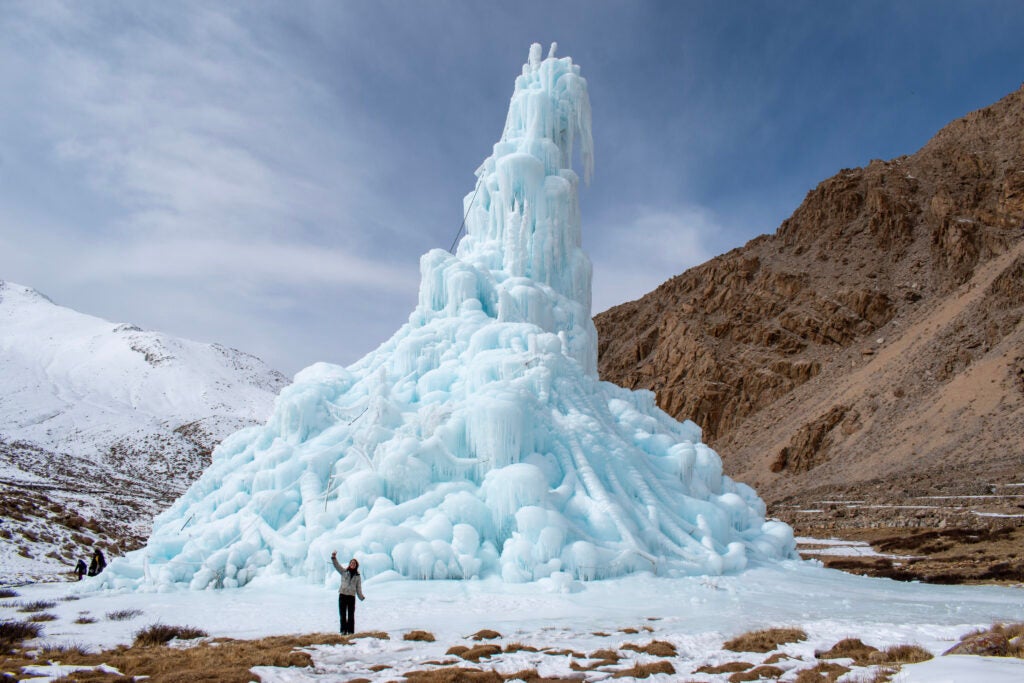
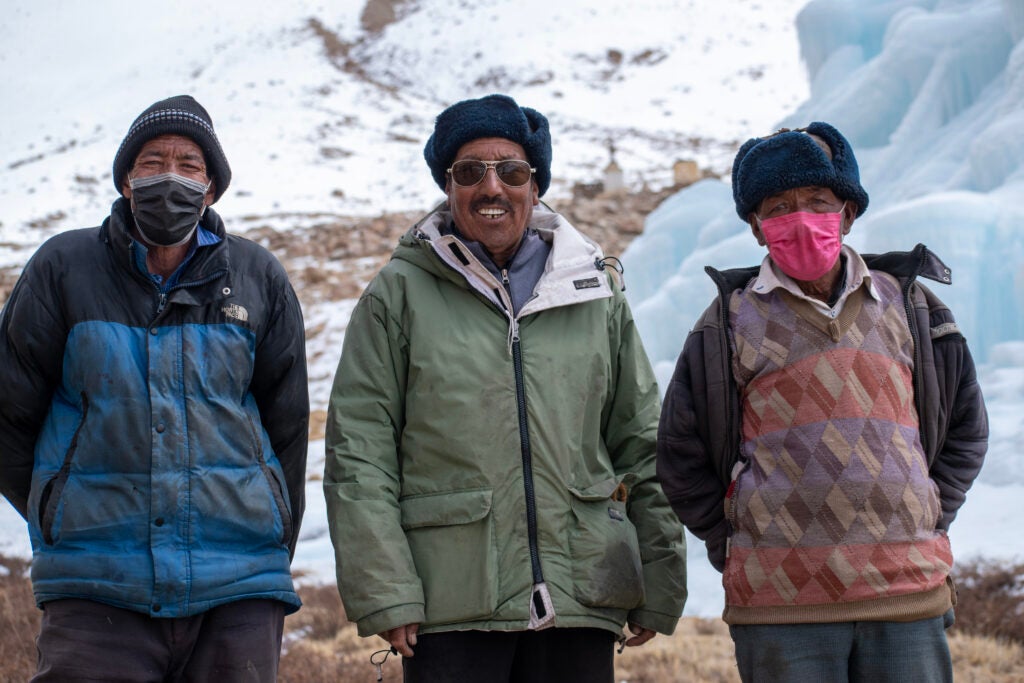
Photo 3: Villagers pose in front of the ice stupa they created in Igoo. Source: Rosa Oyarzún, 2022
Taking inspiration from the design and logic of artificial glaciers, or what might be better described as terraced ice fields, built by a retired civil engineer named Chewang Norphel several decades earlier in Ladakh (Vince 2009), ice stupas store far less water than artificial glaciers. However, their relatively small scale and less invasive presence facilitates construction in a wide variety of environments unlike water tanks and dams.
While artificial glaciers require large, shaded landscapes at specific altitudes, ice stupas can be built at any location that freezes in the winter as long as a feeder stream can be diverted from elevations above. Due to their vertical and conical shape, stupas have a smaller surface area. This means stupas are less exposed to wind and sun compared to horizontal glacial fields.
Wangchuck and his team’s first two-story prototype held 150,000 liters of winter meltwater (Wangchuck 2014). Following this experiment, they were able to garner international support and received funding through a Rolex Award for Enterprise. The funding has allowed the team to construct dozens of ice stupas to supply Ladakhi villages with approximately 25 million liters of water (Maqbool 2019).
One year after learning of the Ladakhi stupas, a group of Chilean engineers began plans to build the first ice stupa prototype in the Andes. They called the project Nilus and recruited an interdisciplinary team to work on the technical model.
In 2021, the team constructed their initial prototype in privately owned Parque Arenas in the Cajón de Maipo, where the waters of the estuaries that feed the Maipo River run off, one of the main sources of fresh water supply for the population of central Chile. The six-meter-high prototype amassed 550,000 kilograms of ice (50,000 liters of water) that melt over 1.8 months (Nilus Presentation April 20, 2022) See Photo 4. This was a preliminary experiment and monitoring exercise, resulting in a limited volume of water.
During winter 2022, faced with a season of heavy snowfall, avalanche conditions, and limited financial resources, the team was able to build a second prototype in the park— although their intention had been to construct three stupas at varying altitudes in order to compare water storage capability and melt time results.
To prevent freezing pipes, which is a common problem for ice stupas, the Nilus team has let the water continually flow and remain in movement. Next winter, they plan to develop ways to remove all the water inside the pipes when the temperature drops below freezing. This will require opening and closing valves, which is not necessarily a sustainable mechanism until automatization has been achieved, given that local populations do not live high in the mountains in Chile.
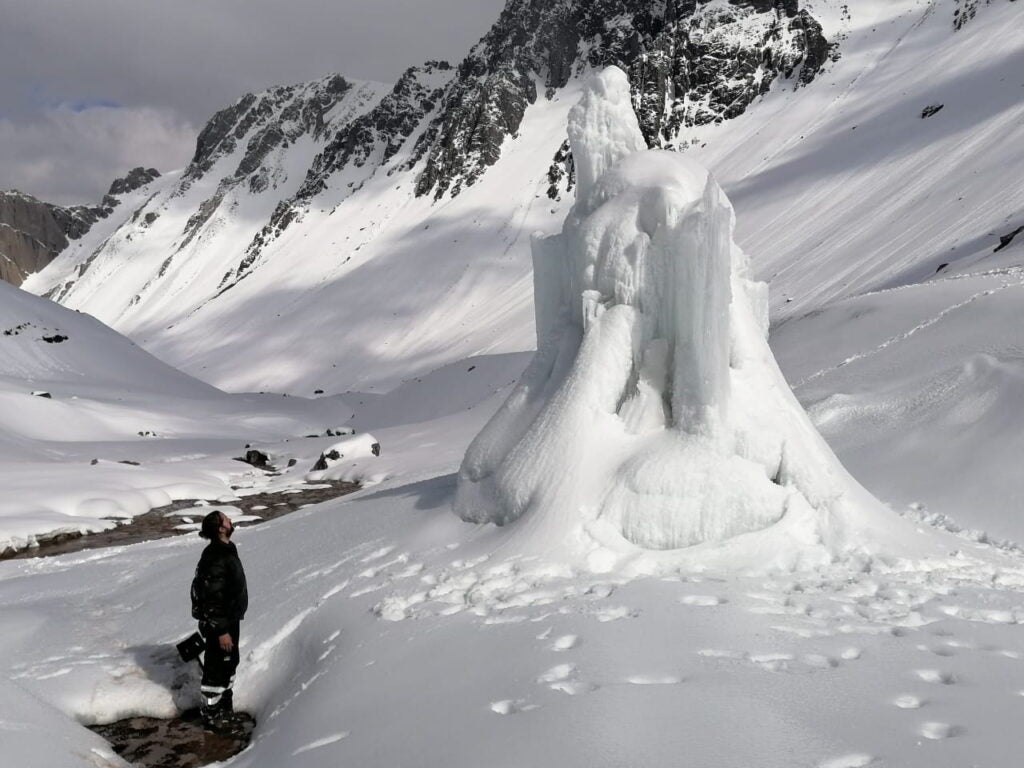
Nilus members have described the Chilean ice stupa project as “combining ancestral Himalayan practices with the development of artificial intelligence (AI)” (Núñez 2022). Their stupas have relied on renewable energy, such as gravity and water pressure, and solar energy to run a climatological station.
The Nilus team aspires to develop a scalable AI model based on geo-hydro-climatic data that will allow them to optimally implement the stupas, as well as to learn in detail the efficiency of their operation. Such data goes beyond altitudinal position to include topographic parameters, such as slope, angle, and solar radiation, as well as tracking the frequency, depth, and duration of fluctuations across the freezing point of water or zero-degree line in winter and spring, along with studying the high mountain ecosystem and interactions of glacier watersheds. Their intention is to eventually produce an automated ice stupa model completely reliant on renewable energy.
In public presentations, members of Nilus have speculated about a range of potential objectives for their project:
- constructing enough ice stupas to buffer glaciers by maintaining a colder microclimate
- restoring high altitude wetlands (bofedales or vegas)
- recovering mountain, foothill, and forest ecosystems
- recovering aquifers using the refrozen meltwater
Currently, their project is less focused on providing water to local downstream populations or becoming an irrigation source. Albeit not incompatible with the Himalayan ice stupas where Wangchuck and his colleagues have focused on “mountain-based solutions for and by mountain-based people” (Interview with Wangchuck on May 25, 2022), the Nilus project’s emphasis is on developing data-driven decision making, remote sensing, and AI. Nilus places emphasis on technological innovation and advanced engineering that would be combined with field measurements. All of this, of course, requires more specialized knowledge, greater resources, and sophisticated technology.
Efficacy and Transferability of Ice Stupas
Despite the climatic and geographic similarities between Ladakh and the central Andes of Chile, there are significant socioeconomic and cultural differences between these two regions. Chile’s privatized water code and the fact that its population does not live at high altitudes in the Andes or necessarily have access to a largely privatized mountain range, creates a particular legal and socioeconomic context within which to experiment with the engineering of ice stupas.
While glacier water cannot be directly owned in Chile, as soon as the ice melts and flows downstream to recharge streams, rivers, and aquifers, this water becomes part of water rights concessions. Privatized water rights add another layer of complexity to the question of who benefits from such emergent infrastructure, as well as the role of technological artifacts in local socio-environmental conflicts over water allocation and other environmental justice issues.
Himalayan ice stupas have not been able to avoid these kinds of conflicts and tensions. Researchers have documented how local communities responded differently to the impacts of the stupas on their customary irrigation governance, especially protests over the perceived abstraction of water or concerns over where the water goes when it enters the high-density polyethylene pipes (HDPE) used to construct the structures (Sharma 2019).
One study conducted by Nüsser et al. (2018) analyzes the efficacy and perceived benefits of different ice reservoirs, mentioning that certain village communities have observed higher crop yields, increased frequency of irrigation, and reforestation. These scholars conclude that “artificial glaciers” should be seen as site-specific water conservation strategies rather than climate change adaptation mechanisms, which they argue, was never their original purpose.
The cultural significance of stupa-like Buddhist shrines, and the fact that the ice stupas are largely community-managed and located close to Himalayan villages, does not necessarily make it easier to deal with freezing pipes and other maintenance issues, climate variability, or natural hazards.
However, it does foment a level of social identification with the projects and community involvement. Rosa Oyarzún, a Nilus member who spent several months learning about the Himalayan “artificial glaciers,” witnessed the way Buddhist ceremonies and female ice stupa climbing competitions cultivated community participation and placed emphasis on the collective and spiritual significance of the stupas beyond their role as simply “water towers” (Interview on March 2, 2022). (See Photo 5).
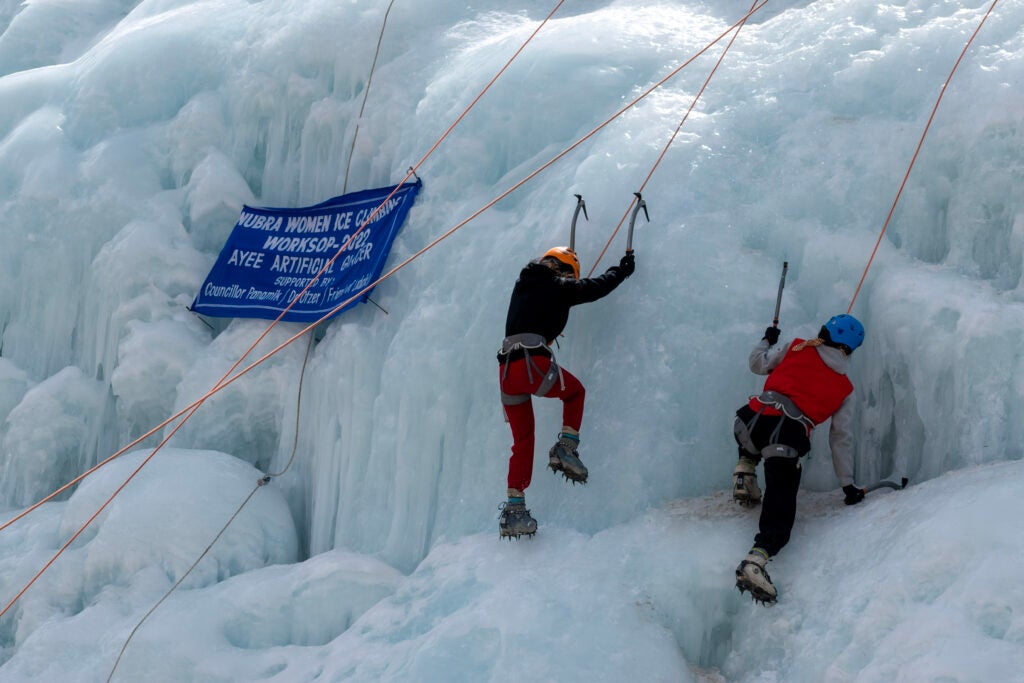
The Nilus project’s prototype is currently located in a private park, and the project has thus far focused on building a model rather than fomenting community involvement. In addition, finding a funding niche to finance the construction of the Chilean ice stupas has proved challenging given that Nilus resembles a startup company and some of its members provide a range of AI services, including services used in industrial mining companies.
The connection to mining has raised red flags for potential donors or funders who identify as environmentalists. Other corporations like Coca Cola, Microsoft, and COPEC (a Chilean energy and forestry company with gas stations throughout the country) have shown financial interest in the project.
Nilus is seeking to first produce a functioning prototype and to produce data about Andean climatic and hydrological conditions, information that still remains largely unavailable for Ladakh and the wider Himalayan region.
In line with Nilus’s focus, some scholars have argued that this information could improve understandings of ice reservoir efficiency, and thus, help evaluate the potential transferability of such water conservation technology.
Recommendations
The reality that both droughts and glacier retreat are increasing means that more and more communities will be affected by water shortage. Beyond water consumption, there are broader consequences of either the disappearance of ice or its replacement by other ecologies.
Engineering projects will continue to be developed to adapt populations and territories to these changes and losses. In their role as potential site-specific water conservation or water harvesting strategies, here are three ideas to consider regarding the engineering of ice stupas and the possible transferability of this technology.
1. Include socioeconomic and historical dynamics
It is important to take seriously the socioeconomic and historical dynamics of human–glacier–water interactions along with the production of geo–hydro–climatic data. It is not advisable to foreground engineering solutions without first probing the structural questions about who needs water, why shortages are occurring, and how legal frameworks and political economic decisions influence access to and allocation of water, protection of glaciers, and conditions of high mountain ecosystems.
In the case of Chile, the fact that glaciers can be located within private property— water is privatized and much of the country’s cordillera is either privatized or, as in the case of the Central Andes, intervened by national and transnational industrial mining operations—requires that projects depending on glacier meltwater acknowledge and study the impacts of the country’s existing legal framework and dominant political economic model.
The location of future ice stupas and decisions over how the water that is stored will be used, and by whom, depend on careful analysis of national, regional, and local contexts if the intention is not only to develop a patentable commercial product for corporate use, but to assist in building solutions that communities can also use in contexts of dramatic ecological and climatic change.
Given that Chileans rejected the results of a national constitutional referendum in 2021 that would have deprivatized water and protected the rights of nature, and glaciers specifically, it is yet uncertain what role ice stupas can play in a regime change in water management and use. Speculatively, the Chilean ice stupas could become an interesting sustainable energy alternative with scalable potential for ecological restoration projects that are implemented in mountainous and foothill regions.
2. Ensure community participation and public access
As scholars have demonstrated, the introduction of new technologies necessarily impacts locally managed institutions, and more broadly, community relations, conviviality, and socio-ecological dynamics. Any project dealing with the allocation of a vital resource, such as water, will (and should) not avoid conflicts, friction, and the need for ongoing dialogue precisely because these are elements of democratic governance, collective decision-making, and participatory processes.
Choosing the site to build an ice stupa or other similar water-harvesting techniques should be done in conversation with local communities, local governance structures, and regional authorities not only to ensure that these projects are compatible with water commons governance, but also to strive for their sustainability in terms of longevity and ability to respond to situated socio-environmental priorities.
Chilean engineering teams that attempt to build ice stupas relying on AI in a context where communities do not inhabit mountainous areas, need to become creative about how they involve nearby and potentially impacted communities at each stage of the project even if the initial focus is not on human water provision, but rather ecological restoration and the replenishing of aquifers.
Ecosystem recovery, and water conservation more generally, deeply implicate downstream communities and a range of different sectors whose livelihoods, recreation, water use, and conservation interests are inseparable from the climatic and ecological conditions of the foothill areas and Andes Mountain range.
3. Structurally situate climate-response engineering
Ice stupas functionally mimic, but do not resemble the complexity and multidimensionality of glaciers and their surrounding ecosystems. Inkpen (2022) has pointed out that “artificial glaciers” underscore an element common to many modifications of the mountain cryosphere. They may reduce glaciers to a functional definition that focuses exclusively on their role as “water towers.” These kinds of projects can also foment the promissory character of technological fixes to climate change.
Other scholars, and many activist organizations and networks, have pointed out that technological interventions tend to draw attention away from the anthropogenic causes that concern the geopolitical, structural, and historical processes provoking climate alteration, and with this, retreating glaciers. Indigenous scholars, in particular, have argued that diminishing ice provokes colonial acceleration and further deferment of climate justice for Indigenous peoples (Whyte (2020).
Without wanting to foreclose the potentiality of ice stupas to become more than site-specific water conservation strategies, it is important to pursue climate adaptive engineering projects while acknowledging their limitations and working to address the root causes of the need for their development.
This is especially pertinent when it comes to eliminating immediate anthropogenic interventions in glacier ecosystems, such as road building, mining operations, hydroelectric dam construction, intense tourism (including mechanized vehicles), and other forms of direct and indirect impact on high mountain ecosystems.
This paper is published in collaboration with The Water Center at Penn. Special thanks to academic reviewer Tony Sauder, senior advisor at The Water Center, members of the Nilus project, especially Manuel Soto, Sebastián Goldschmitt, and Rosa Oyarzún, and also Gustavo Rebolledo.
Kristina Lyons
Assistant Professor of AnthropologyKristina Lyons is an assistant professor of anthropology in the School of Arts and Sciences and the Penn Program in Environmental Humanities (PPEH) at the University of Pennsylvania.
Budds, J. 2009. “Contested H2O: Science, Policy and Politics in Water Resource Management in Chile,” Geoforum: Journal of Physical, Human, and Regional Geosciences 40(3): 418-430.
Carey, M., J. Barton, and S. Flanzer. 2022. “Glacier Protection Campaigns: What Do They Really Save?” Ice Humanities: Living, Working, and Thinking in a Melting World. Edited by Klaus Dodds and Sverker Sörlin, 89-109. Manchester, England: Manchester University Press.
Carey, M. 2010. In the Shadow of Melting Glaciers: Climate Change and Andean Society. Oxford: Oxford University Press.
CIVICUS. 2020. “Chile Has Entirely Privatized Water, Which Means That Theft is Institutionalized.” https://www.civicus.org/index.php/media-resources/news/interviews/4271-chile-has-entirely-privatised-water-which-means-that-theft-is-institutionalised, February 20.
Clouse, C. 2016. “Frozen Landscapes: Climate-Adaptive Design Interventions in Ladakh and Zanskar.” Landscape Research 41(8): 821-837.
CR2. 2015. Report to the Nation. The 2010–2015 Mega-Drought: A Lesson for the Future. www.cr2.cl/megasequia.
Crespo, S. A., C. Lavergne, F. Fernandoy, A. Muñoz, L. Cara, and S. Olfos-Vargas. 2020. “Where Does the Chilean Aconcagua River Come From? Use of Natural Tracers for Water Genesis Characterization in Glacial and Periglacial Environments.” Water 12: 2630.
Cruikshank, J. 2005. Do Glaciers Listen? Local Knowledge, Colonial Encounters, and Social Imagination. Vancouver: University of British Columbia Press.
Dirección General de Aguas (DGA). 2022. Inventario Público de Glaciares. https://dga.mop.gob.cl/Paginas/InventarioGlaciares.aspx.
Dodds, K., and S. Sörlin. 2022. Ice Humanities: Living, Working, and Thinking in a Melting World. Manchester, England: Manchester University Press.
Dussaillant, I., E. Berthier, F. Brun, M. Masiokas, M., R. Hugonnet, V. Favier. 2019. “Two Decades of Glacier Mass Loss Along the Andes.” Nature Geoscience 12: 802-808.
Gagné, Karine. 2016. “Cultivating Ice over Time: On the Idea of Timeless Knowledge and Places in the Himalayas.” Anthropologica 58(2): 193-210.
Garreaud, R.D.; J.P. Boisier, R. Rondanelli, A. Montecinos, H.H. Sepúlveda, D.Veloso-Aguila. 2020. “The Central Chile Mega Drought (2010–2018): A Climate Dynamics Perspective.” International Journal of Climatology 40: 421–439.
Hernando-Arrese, M., and M. Tironi. 2019. “Worlding Hydropower: River Realities in the Chilean Patagonia.” Tapuya: Latin American Science, Technology and Society 2(1): 295-309.
Inkpen, D. 2022. “Ever Higher: The Mountain Cryosphere.” Ice Humanities: Living, Working, and Thinking in a Melting World.” Edited by Klaus Dodds and Sverker Sörlin, 72-88. Manchester, England: Manchester University Press.
IPCC. 2019. Special Report on the Ocean and Cryosphere in a Changing Climate. Accessed October 8, 2022. https://www.ipcc.ch/srocc/.
Krupnik, I., C. Aporta, S. F. Gearhead, G. J. Laidler, and L. K. Holm (eds). 2010. SIKU: Knowing Our Ice: Documenting Inuit Sea-Ice Knowledge and Use. New York: Springer.
Maqbool, R. 2019. “In Kashmir, an Ancient Solution Solves a New Problem.” Global Press Journal, January 13.
Masiokas, M.H., A. Rabatel, A. Rivera, L. Ruiz, P. Pitte, J.L. Ceballos, G. Barcazo, A. Soruco, F. Brown, E. Berthier, I. Dussaillant and S. MacDonell. 2020. “A Review of the Current State and Recent Changes of the Andean Cryosphere.” Frontiers in Earth Science 8:99: 1-27.
Núñez, R. 2022. “Proyecto Nilus: un laboratorio del agua en los Andes Chilenos.” El Ágora: diario del agua. https://www.elagoradiario.com/agua/proyecto-nilus-agua-andes-chilenos/
Nüsser, M., and R. Baghel. 2016. “Local Knowledge and Global Concerns: Artificial Glaciers as a Focus of Environmental Knowledge and Development Interventions,” in Ethnic and Cultural Dimensions of Knowledge: Knowledge and Space. Edited by P. Neusburger, T. Freytag, and L. Suarsana, 191-209. Switzerland: Springer.
Nüsser, M.; J. Dame; B. Kraus; R. Baghel, and S. Schmidt. 2018. “Socio-Hydrology of ‘Artificial Glaciers’ in Ladakh, India: Assessing Adaptive Strategies in a Changing Cryosphere.” Regional Environmental Change 19:1327-1337.
Pena, H., and B. Nazarela. 1987. “Snowmelt-Runoff Simulation Model of a Central Chile Andean Basin with Relevant Orographic Effects,” IAHS-AISH 166: 161-172.
Schmidt, S., and M. Nüsser. 2017. “Changes of High-Altitude Glaciers in the Trans-Himalaya of Ladakh over the Past Five Decades (1969-2016).” Geosciences 7:27.
Sharma, A. 2019. “Giving Water Its Place: Artificial Glaciers and the Politics of Place in a High-Altitude Himalayan Village.” Water Alternatives 12(3): 993-1016.
Tamayo Grez, T. and A. Carmona López. 2019. El Negocio del Agua: Cómo Chile se Convirtió en Tierra Seca. Santiago: Penguin Random House.
United States Department of Agriculture Foreign Agricultural Service. 2022. Modernization of Chile’s Water Code: Report Number CI2022-0002. https://apps.fas.usda.gov/newgainapi/api/Report/DownloadReportByFileName?fileName=Modernization%20of%20Chile%27s%20Water%20Code_Santiago_Chile_01-18-2022.pdf
Valdés-Pineda, R., R. Pizarro, P. García-Chevesich, J. Valdés, C. Olivares, M. Vera, F. Balocchi, F. Pérez, C. Vallejos, R. Fuentes, A. Abarza, and B. Helwig. 2014. “Water Governance in Chile: Availability, Management and Climate Change.” Journal of Hydrology 519: 2538-2567.
Vince, G. 2009. “Glacier Man.” Science 326(5953): 659-661.
Wangchuck, S. 2014. “Ice stupa artificial glaciers of Ladakh.” https://www.indiegogo.com/projects/ice-stupa-artificial-glaciers-of-ladakh
Whyte, K. 2020. “Too Late for Indigenous Climate Justice: Ecological and Relational Tipping Point,” WIREs Climate Change 11: e603.