Energy and the Blockchain: Opportunities and Challenges for Climate and Energy Governance
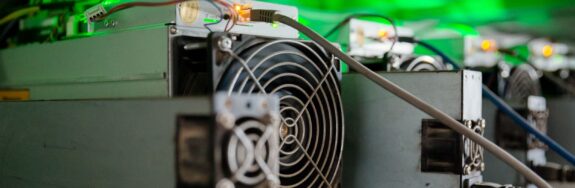
With the rise of Bitcoin and other blockchain networks, there is growing interest in using this peer-to-peer verification technology to the energy industry—potentially revolutionizing the way we generate and distribute energy and monitor emissions.
At the Annual Consumer Electronics Show this year, the camera and photography company Kodak made a surprise announcement. At the end of January, 2018 they launched KodakOne, a new blockchain-based network—essentially an internet network that allows many servers to share and replicate accounting ledgers—complete with its own cryptocurrency, KodakCoin (Sussman 2018). Despite remaining uncertainty about how exactly this platform will benefit photographers, the announcement sent the struggling corporation’s stock soaring from $3.10 on January 8, to $10.70 on January 10. Subsequently, it has dropped back to a value of approximately $6.00 over the last several weeks. This one recent event exemplifies the wave of excitement around blockchain technology that swept up global investors in recent months. Some of this excitement concerns the technology’s application in energy markets, as is the focus of this digest.
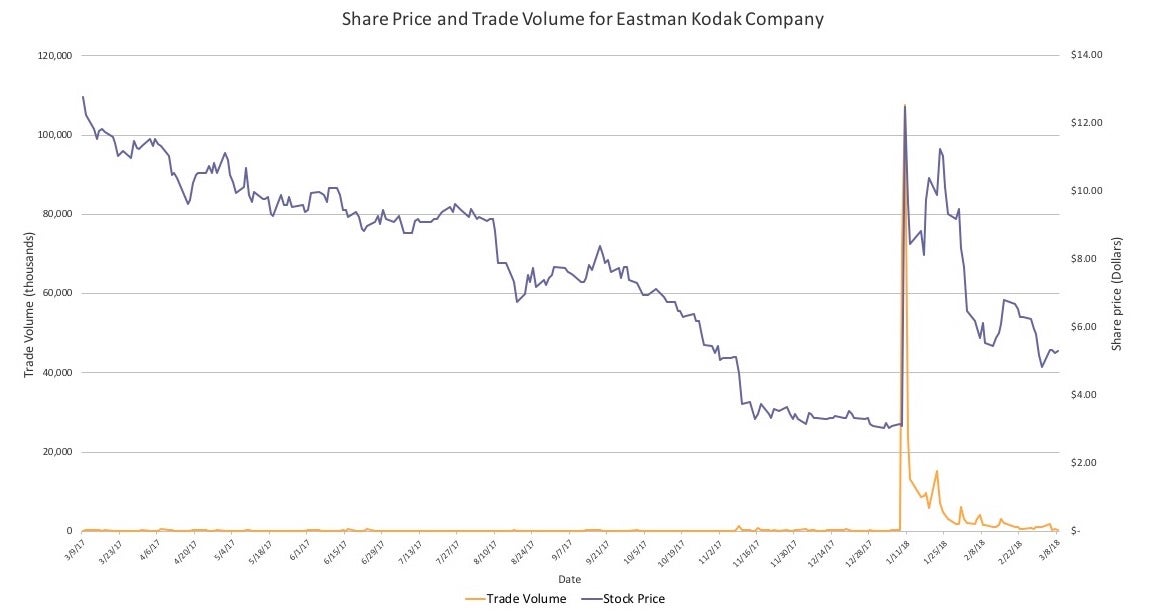
In all likelihood, you have recently encountered the word “blockchain” in relation to coverage of Bitcoin. The reason Bitcoin has been in the spotlight is primarily because of the astonishing volatility of its value. Over the course of 2017, Bitcoin’s value surged by approximately 2,000%, and has subsequently dropped back to about 50% of its peak value (coinmarketcap.com). Stories of overnight billionaires and popping market bubbles have left the rest of us to ponder how we seemingly missed out on what appears to have been a $300 billion internet free-for-all (Popper 2017).
It is important to note that the value of bitcoins, and of hundreds of other cryptocurrencies, indicates a growing interest in the technology, but only marginally impacts the subsequent discussion of how this technology can and should be used and regulated. This energy policy digest will describe exactly what the blockchain is, explore several common critiques of this technology, and draw conclusions about how this technology will likely impact climate and energy governance.
What is a Blockchain?
Within the World Wide Web, a website (a manifestation of data and/or computer code) is stored on centralized servers, and you, as a consumer, are able to interact with that data using a URL address and web account. When you go on Amazon and order an item, for example, your computer does not store a record of that transaction. That transaction is stored, processed, and completed by the Amazon servers. You are only comfortable making that purchase because you trust the integrity and security measures of the company responsible for housing those servers. You trust that Amazon will email you a confirmation email, that the order will be completed, and that the item will arrive at your door in a matter of days. However, if that order was somehow compromised on the Amazon server, you would have very little proof of ownership.
Similarly, if you log on to your bank account, you can see how much money you own, but in reality that ownership is simply a digital agreement between you and your bank, housed on their centralized servers. If your bank’s account ledgers were tampered with, or if your bank goes bankrupt, your funds could be lost. While it may be that the bank or online store is at fault, your ability to recover your property may depend on the company’s policy, your ability to take legal action, or the laws of the country where you live.
Blockchains, simply put, are open network protocols that decentralize the storage of data so it is highly redundant, independent of any single authority, theoretically tamper-proof, and, in the case of international transactions, often faster than traditional bank transfers (IBM Think Academy 2016). This speed and security is achieved by the network independently verifying each transaction by solving a highly randomized algorithmic equation, often requiring astonishing quantities of computational power to solve.
A record of these verified transactions is simultaneously housed across thousands of servers in the blockchain network, each verifying the communal ledger. Even if a single node of this ledger gets hacked, the system will self-correct any erroneous data that is not verified by the majority of other nodes within the network. This means that you can be highly certain that no one will question your ownership of the digital asset (e.g. bitcoins) that you have purchased.
A record of every transaction ever made on a blockchain (a.k.a. distributed ledger) network is publically viewable and practically impossible to edit once verified. This feature of blockchain protocols makes them highly transparent, and a powerful tool in tackling fraud, corruption, and double-counting. If a dispute arises over asset ownership, there is a tamperproof record viewable to anyone within the network.
Another useful feature of blockchain protocols is that they function completely autonomously. No one owns the blockchain network, and it doesn’t require a centralized administrative authority; only well-scripted, periodically updated code. In the case of cryptocurrencies, this is often touted as a major advantage over state-issued currencies which can be heavily influenced by a government’s decision to print additional currency, leading to currency inflation (Gavril 2017). As an example, the Bitcoin network will only ever allow 21 million bitcoins to be issued.
Exactly how a blockchain transaction gets verified depends on the blockchain network. In the case of Bitcoin, and other “proof-of-work” protocols, groupings of transactions, called blocks, are verified by “miners”; groups that have invested in highly specialized computer hardware, capable of solving these equations very rapidly. These miners compete to be the first to solve an individual block of transactions, in exchange for a payment of the network’s digital currency if they win. This reward amounts to a small fraction of new bitcoins entering the network, leading to an asymptotically decreasing growth in the total number of bitcoins.
Energy and the Blockchain
Along with the recent speculative wave of interest in blockchain technologies and cryptocurrencies, there has also been significant discussion of how this technology could impact how we manage everything from personal data privacy, to energy generation, distribution, and emissions. In his recent New York Times Magazine article, Steven Johnson goes as far as to suggest that blockchain networks may help to disassemble the tech monopolies that have emerged over the last two decades such as Uber and Facebook. Several of the largest cryptocurrency networks such as IOTA and Power Ledger emphasize how their networks can be used in partnership with the internet of things and sources of distributed energy generation such as rooftop solar, vehicle charging stations, etc. (Popov 2017 & Power Ledger 2017).
The picture these network organizations paint is one of a near future where appliances, solar cells, and vehicles could communicate with each other and send automatic payments to each other using a blockchain network. If a distributed solar installation was generating more electricity than the owner was using, it could communicate with nearby energy users and sell that electricity via payment transactions on the blockchain. It is not a stretch to envision how this network could easily be expanded to accommodate payments of other energy related services such as battery storage and carbon credits.
On paper, this sounds ideal. Peer-to-peer energy transactions could improve grid efficiency, and incentivize consumers to invest in efficient and clean technologies. In March, Green Tech Media is hosting an energy forum on blockchain, exemplifying the industry’s interest in pursuing this vision of the future (Green Tech Media 2017, Basden & Cottrell 2017). However, a deeper exploration of these goals is warranted to determine the likely costs and benefits.
Energy Demand
What makes blockchain networks so revolutionary is that every transaction, or change in ownership of digital property, is verified using extremely complex algorithms. This provides users with a high level of security, but it does come at a cost; specifically, an energy cost. The amount of energy that is consumed by the Bitcoin network is difficult to know for certain because of a constantly fluctuating demand and ever-increasing verification complexity. However, best estimates put the annual usage at between 32 and 34 TWh, or 250 KWh per block verification—roughly equivalent to one week of electricity consumption by the average American household (Energy Information Administration 2017). It is estimated that during the recent price spike of bitcoins, energy demand was increasing by 450 GWh (or approximately 250,000 barrels of oil) every day.
At this rate, some estimates suggest that Bitcoin and other cryptocurrencies that use the same high energy “proof-of-work” protocol could significantly shift future estimates of worldwide energy use and related carbon emissions (E&E News 2018, Irfan 2017). There is evidence that this new demand for energy is already contributing to blackouts in countries with vulnerable infrastructure-such as Venezuela where people are turning to Bitcoin in the face of Bolivar hyperinflation (Grist 2017).
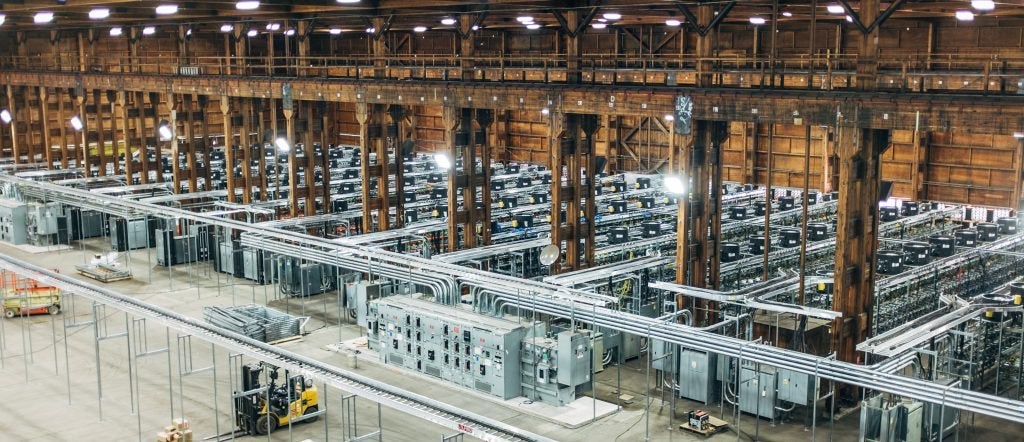
Given the enormous and rapidly increasing energy demand of blockchain technology, the suggestion that decentralized ledger networks could provide any tangible benefits to energy and climate governance seems misinformed and possibly counter-productive. Indeed many journalists are sounding the alarm over this “malignant” technology (Grist 2017). At the same time, others are suggesting that the future energy demand of “proof-of-work” blockchains is overstated.
One such argument is that, while the energy cost of mining bitcoins is high, it is not as high as printing or minting physical currency (Schultz 2018). One must also consider, however, that far more physical currency is printed than bitcoins, and that the majority of U.S. dollars in circulation today are digital, rather than physical. Another counter argument to Bitcoin being too energy intensive suggests that this will not be an issue as long as bitcoin value experiences only modest growth (Gimon 2018). If bitcoin’s value continues to rise, the reward a miner receives also holds more value, and thus, he/she can afford to use more energy to attempt to solve a block algorithm. The problem with this argument is that the Bitcoin blockchain is designed so that there will never be more than 21 million bitcoins in circulation. Therefore, as with any limited resource, if demand for the digital coin goes up, the price will increase as well.
Should demand for bitcoins increase, it is likely that the computational mining power and energy demand of the network will increase proportionally. That tends to rule out any suggestion that the Bitcoin network is sustainably scalable, or that it is capable of incorporating such transactions as distributed energy payments. That being said, Bitcoin, and the network it is built on, is not the only form of blockchain technology that exists.
Alternatives to Bitcoin
There are over 4,000 cryptocurrencies currently in circulation, with more emerging every day; and dozens of these networks were specifically designed to address the scalability issues of the Bitcoin network.
Cryptographic strategies for block verification include a “proof-of-stake” protocol and Directed Acyclic Graph protocols, among others. “Proof-of-stake” network participants are able to verify transactions based on their ownership of the network’s cryptocurrency (or other digital asset), rather than by competing with each other to solve a block algorithm. This protocol, in theory, should vastly reduce the energy consumption of the network, and should allow a wider audience of users to take part in the verification-or “mining”-process.
There are, however, concerns that this protocol may not provide the network security that “proof-of-work” networks like Bitcoin can offer. Directed-acyclic-graph protocols, such as the IOTA network, are designed so that each transaction cannot be completed until the participants in that transaction verify at least two previously completed transactions. In this way, previously executed transactions are independently verified by a number of later transactions, using a fraction of the computing power of other verification methods.
It is currently unclear which, if any, of these alternative networks has successfully built a secure and energy efficient protocol. Theoretically the energy demand of Bitcoin is a design flaw of a verification process which allows multiple miners to compete against each other with computing power. However, this is not intrinsic to blockchain technology as a whole.
It is likely that an alternative network protocol could offer widespread applications in energy governance without massively increasing global energy demand. That being said, there are other barriers to the use of blockchain networks in energy management and grid transactions. These issues are not an inherent weakness of blockchain technology, but rather an inability of the protocol to address several of the problems that energy utilities are currently facing.
Who Pays for Grid Maintenance?
Traditionally, utilities would maintain and operate a distribution network while customers who were connected to that network would consume energy through a unidirectional electricity meter and pay the utility a proportional monthly fee. However, with the rise of private and community-owned distributed generation, utilities are facing a pressing challenge. They still need to maintain the distribution network to a customer’s home, but that customer may only require grid electricity on days when solar irradiation is insufficient to meet their needs, and therefore their monthly bill will not meet the utilities’ costs of maintaining the distribution infrastructure that services that customer.
Distributed energy pricing programs such as net metering further strain utility revenues, obligating the utility to purchase excess solar power from homes, even if current load is sufficient to meet demand. This accentuates load variability over the course of the day, and further reduces utility revenue. An alternative pricing structure that charges the consumer separately for their energy usage and for their grid connection could help solve many of these system vulnerabilities.
It is not clear how a distributed ledger system would necessarily remedy the issue of utility revenue, which is caused by unsustainable and outdated pricing structures. Peer-to-peer transactions would certainly improve grid efficiency, but would only work if the communally used distribution infrastructure is maintained. One could theoretically envision a network where a share of every blockchain transaction was given to the utility responsible for maintaining the distribution network, but there is little to suggest that this would be sufficient to cover maintenance and network oversight costs.
Alleviating Load Variability
Even if distributed solar generation were able to meet most demand at certain times of the day, there will always be times when baseload, grid-scale electricity is needed. This imbalance in generation capacity may lead to an energy surplus during daytime hours, and high demand for grid electricity during the evening.
To address this load variability, utilities will either need to invest heavily in distribution-level battery storage, or there will need to be a major increase in fast-ramping grid-level baseload generation. Either of these situations will likely require high institutional investment. Peer-to-peer blockchain transactions between grid-connected customers bypasses the utility and may therefore reduce institutional revenue, at least under existing pricing schemes (Lielacher 2017). Removing this flow of cash from the utility would make investing in grid level infrastructure more difficult.
In short, while distributed ledger transaction networks in theory sound well-positioned to become the default method of building local distributed energy markets, it is not clear that this technology actually helps to solve any of the most pressing challenges facing distribution utilities. Until such time as blockchain networks demonstrate themselves to be more efficient than utility managed payments, or to lend themselves to solving utility challenges that standard payment structures cannot solve, it is difficult to see any immediate demand for the application of this technology to distribution level energy payments.
Blockchain Cap and Trade
Customer-to-customer blockchain payments offer uncertain benefits to electricity utilities; however, there are other areas of energy governance where blockchain networks may be more readily applicable. Cap-and-trade programs are most often designed to provide industry with an incentive to reduce carbon emissions. This is done by a governing body agreeing to the total quantity of industrial carbon that can be emitted within the jurisdiction. That pre-determined quantity is represented by a number of equally weighted carbon credits, or allowances, which are distributed to industrial emitters, usually by free allocation and auctions. Funding that the government receives from these auctions is typically reinvested into clean energy efforts.
Any facility that produces more carbon emissions than its existing credits will allow, is required to purchase unused credits from another facility through a secondary market. These programs have been successfully implemented around the world, in many European countries and, by the end of this year, in China, the country with the highest overall carbon emissions (Buckley 2017). This method of emissions governance is not, however, free from criticism. Many argue that a carbon tax is a far more transparent and stable method of putting a price on carbon (World Resources Institute). In the United States, there are two programs that can demonstrate some of the strengths and weaknesses of cap-and-trade initiatives: The Regional Greenhouse Gas Initiative (RGGI), and the CARB cap-and-trade program in California.
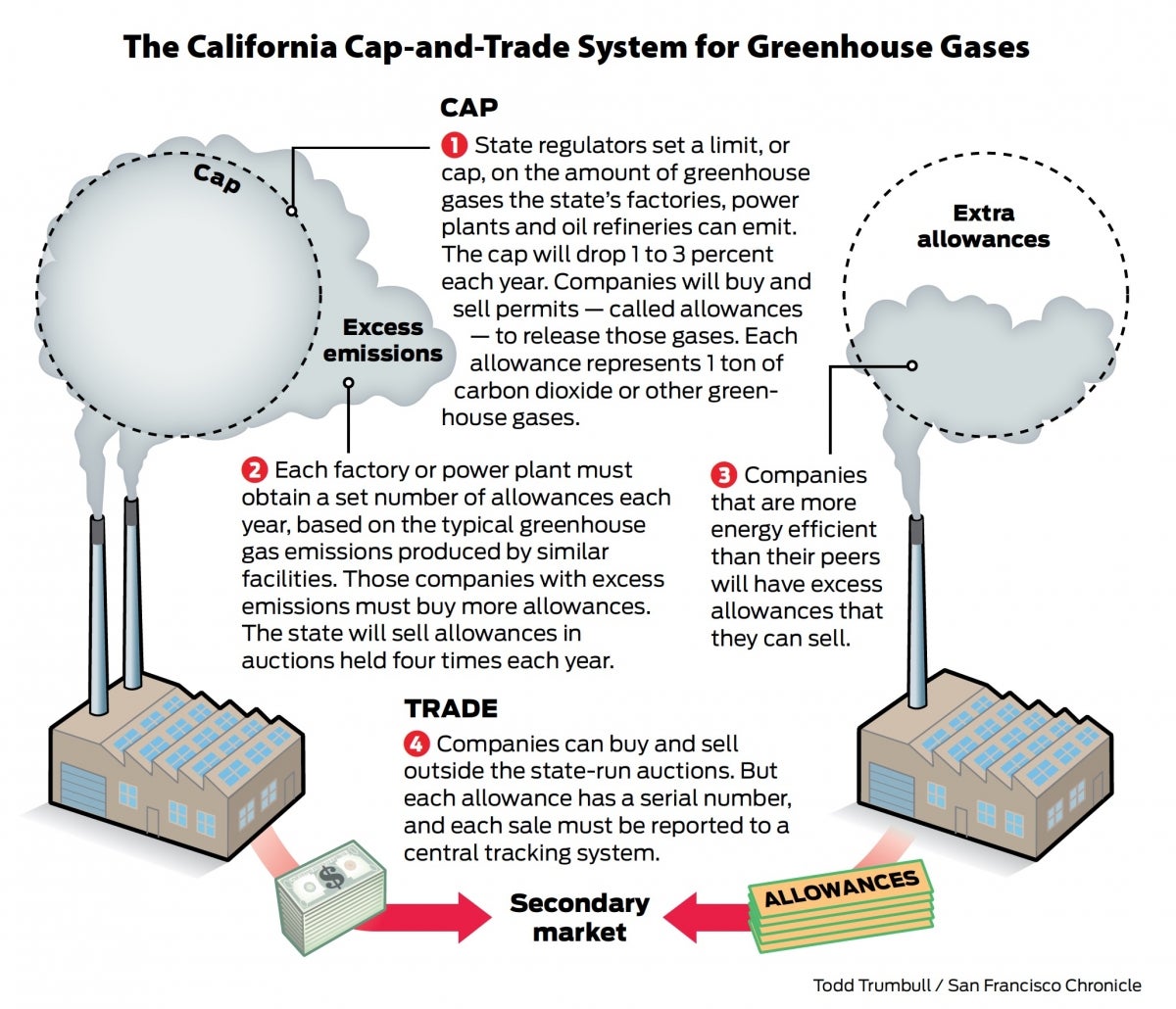
Distributed ledger technology may offer a highly applicable solution to some of the criticisms that cap-and-trade programs have faced over the years, and that continue to be raised by critics of both RGGI and the CARB cap-and-trade program (Walker 2017).
Transparency
Cap-and-trade programs are often criticized for concealing the cost of carbon that society is paying. For better or worse, a cap-and-trade system is a somewhat more complicated and opaque method of achieving the same results as a straightforward carbon tax. This can make cap-and-trade policy slightly more palatable to constituents who are opposed to any kind of tax increases, but it also makes these programs much more difficult for the public to monitor. Double-spending, collusion, price manipulation, allowance hoarding, and false reporting are all serious concerns when it comes to cap-and-trade programs. As a result, they require oversight by an independent market monitor (RGGI 2017).
By transferring carbon allowances to a distributed ledger or “blockchain” network, purchases and trades between businesses and state or regional government would be viewable to anyone with an internet connection. This would allow journalists and concerned constituents to monitor trade patterns within the industry without relying on quarterly reports published by a centralized authority. As on-site monitoring of emissions becomes increasingly affordable and legislatively supported, these data can easily be integrated into a distributed ledger system through the use of “smart contracts”; programs that run on the network and demand certain criteria to be met before a transaction takes place (Coindesk 2017). Having an automatically updated and publically available record of credits also provides program analysists with a much clearer picture of the success of a carbon trading program. Analysists can see, at any point, exactly how many tons of carbon have been paid for by program participants.
Credit Expiration and Hoarding
As with any tradable item, virtual or otherwise, carbon credits are inherently susceptible to market manipulation and hawkish behavior. In the absence of regulation preventing such behaviors, businesses can purchase more credits than necessary while the price is low, and sell them while the price is high. In extreme cases, a single buyer of credits could manipulate the market price for credits by artificially restricting the supply. Under existing U.S. programs, these vulnerabilities are typically managed by limiting the percentage of auctioned allowances that any single buyer can purchase, and by limiting the number of allowances a business can “bank” for later use (Roberts 2017, C2ES 2017).
However, this regulatory strategy has its weaknesses. If market participants are allowed to store a significant number of credits, they create a market surplus of credits and consequently higher than intended emissions. Using a decentralized ledger platform to maintain a cap-and-trade system, there is an alternative method of preventing hoarding and market manipulation. Using block chain technology, it is possible for each token to carry with it unique characteristics, say for example, an expiration date. That token could be traded or used up until the expiration date, effectively disappearing henceforth. As tokens expire, an equivalent number of tokens could be automatically distributed to carbon neutral or negative businesses, maintaining a consistent, or slowly decreasing, market supply of tokens, again with no necessary government oversight.
Market Scalability
One of the major benefits of a distributed ledger crypto-governance model for cap-and-trade programs is the inherent market scalability of the system. Say, for example, California began using a blockchain network to administer their cap-and-trade protocol, this would create an open market for industrial facilities within the state to buy and sell carbon tokens. If Washington state then decided to pass a cap-and-trade law, it could reach an agreement with California to allow Washington based industrial facilities to discount their carbon emissions using tokens from the California blockchain network. This could be done by issuing new tokens via free distribution to carbon negative organizations, proportional to the target carbon emissions goals of Washington state. This expansion of the ledger network would not require any rewriting of the underlying protocol, nor any cumbersome expansion of oversight.
Conclusions
It is unclear exactly how the use of blockchain technology will evolve in the coming years. Current speculation over the value of cryptocurrencies has sent this emergent technology industry on a vastly unpredictable rollercoaster ride, and excitement over the technology seems to be clouding judgment of how best to apply it.
However, distributed ledger networks clearly offer important advantages over traditional bank transactions in the form of increased flexibility, security, transparency, and speed. In instances of energy and climate governance where these benefits are particularly relevant, it is almost certain that blockchain networks will eventually become the default system. In the case of distribution level customer-to-customer transactions, however, there has yet to be a clear demonstration of what advantages blockchain technology has over a utility-managed customer-to-customer bank payment system, or how this emerging technology helps to finance grid infrastructure in a distributed electricity market.
The author wishes to acknowledge the helpful comments of Dr. James R. Hines. Any remaining errors are the responsibility of the author alone.
Oscar Serpell
Deputy DirectorOscar Serpell oversees all student programming, alumni engagement, faculty and student grants, and visiting scholars. He is also a researcher, writer, and policy analyst working on research initiatives with students and Center partners.
Basden, James & Cottrell, Michael. 2017. How Utilities Are Using Blockchain to Modernize the Grid. Harvard Business Review. https://www.governmentblockchain.org/wp-content/sabai/File/files/ff6fe78d6a47e138f7bc41c2bb824c2b.pdf
Buckley, Chris. 2017. Xi Jinping Is Set for a Big Gamble With China’s Carbon Trading Market. The New York Times.
http://nyti.ms/2t1XBf7
Energy Information Administration. Frequently Asked Questions: How Much electricity does an American home use?
https://www.eia.gov/tools/faqs/faq.php?id=97&t=3
Center for Climate and Energy Solutions. California Cap and Trade.
https://www.c2es.org/content/california-cap-and-trade/
Climatewire: Finance. 2018. Bitcoin will demand more energy than EVs – analysts. E&E News.
https://www.eenews.net/climatewire/2018/01/12/stories/1060070823
Cryptocurrency Market Capitalizations: Bitcoin. Coinmarketcap.com.
https://coinmarketcap.com/currencies/bitcoin/
Gavril, Matei. 2017. The Financial Revolution And The Many Benefits It Brings: Cryptocurrency & Blockchain Technology. Forbes. https://www.forbes.com/sites/forbescommunicationscouncil/2017/11/15/the-financial-revolution-and-the-many-benefits-it-brings-cryptocurrency-blockchain-technology/#4b8fef063cc0
Gimon. Eric. 2018. Bitcoin Mining’s Energy Use Won’t Eat The World – If Prices Stay Below 19% Annual Growth. Forbes. http://bit.ly/2nuHZfM
Green Tech Media Events: Blockchain in Energy Forum. Greentechmedia.com
https://www.greentechmedia.com/events/live/blockchain-in-energy-forum#gs.B1cADb0
Holthaus, Eric. 2017. Bitcoin could cost us our clean-energy future. Grist.org.
https://grist.org/article/bitcoin-could-cost-us-our-clean-energy-future/
How Do Ethereum Smart Contracts Work? Coindesk.com. http://bit.ly/2xqOhQU
Irfan, Umair. 2017. Bitcoin’s price spike is driving an extraordinary surge in energy use. Vox.com
https://www.vox.com/energy-and-environment/2017/12/2/16724786/bitcoin-mining-energy-electricity
Johnson, Steven. 2018. Beyond the Bitcoin Bubble. The New York Times Magazine.
https://www.nytimes.com/2018/01/16/magazine/beyond-the-bitcoin-bubble.html
Kaufman, Noah. 2016. Carbon Tax vs. Cap-and-Trade: What’s a Better Policy to Cut Emissions? World Resources Institute.
http://bit.ly/1TlNicR
Lielacher, Alex. 2017. How The Blockchain Can Create A True Peer-To-Peer Sharing Economy. Nasdaq.
http://bit.ly/2t2txzx
Market Monitor Reports. The Regional Greenhouse Gas Innitative.
https://www.rggi.org/auctions/market-monitor-reports
Popov, Serguei. 2017. The Tangle. Iota.org. https://iota.org/IOTA_Whitepaper.pdf
Popper, Nathanial. 2017. How the Winklevoss Twins Found Vindication in a Bitcoin Fortune. New York Times.
https://www.nytimes.com/2017/12/19/technology/bitcoin-winklevoss-twins.html
Power Ledger. 2017. White Paper. Powerledger.io.
https://powerledger.io/media/Power-Ledger-Whitepaper-v3.pdf
Roberts, David. 2017. California is about to revolutionize climate policy … again. Vox.
https://www.vox.com/energy-and-environment/2017/5/3/15512258/california-revolutionize-cap-and-trade
Schultz, Ben. 2018. The energy toll of cryptocurrencies is overstated, and here’s why… Energy Central http://bit.ly/2BMFkm2
Sussman, Bob. 2015. The return of cap and trade is good news for U.S. climate policy. Brookings. http://brook.gs/2s24w8i
Sussman, Louie. 2018. Uncertainty Dampens Hopes That Kodak’s Cryptocurrency Can Help Photographers Get Paid. Artsy.net
https://www.artsy.net/article/artsy-editorial-uncertainty-dampens-hopes-kodaks-cryptocurrency-help-photographers-paid
Walker, Lisa. 2017. This new carbon currency could make us more climate friendly. World Economic Forum.
http://bit.ly/2xmpxLB
Walsh, Bryan. Why the Climate Bill Died. TIME. http://science.time.com/2010/07/26/why-the-climate-bill-died/
Video: IBM Think Academy: Blockchain, How it works. 2016. IBM Think Academy.
https://www.youtube.com/watch?v=lD9KAnkZUjU