Economics and Policy of Large-Scale Battery Storage

If the share of solar and wind generation on the electric grid is to become large, the remainder of the grid must improve in its ability to handle peaks and valleys in demand. A host of technologies exist to deal with mismatched supply and demand, for example natural gas turbines that can be switched on quickly when demand is high, or systems to pump water into reservoirs during low-demand periods and release it (back through electric turbines) during high-demand periods.1
In recent years a new and promising competitor has emerged: battery storage. A thorough engineering literature now exists on creating large-scale batteries, spanning sodium-sulphur, lithium ion, lead-acid, and more. But comparatively little has been written about the economics of large-scale batteries.
In the digest that follows, I first review a set of recent papers in the economics literature, and then step back to consider a broader perspective: How do some of the fundamental lessons from environmental economics apply to the challenge of adapting our electric grid? What lessons can we take from existing policy to guide the use of incentives or mandates for battery technology?
Studies in Economics
Richard Carson and Kevin Novan approach the question of battery storage directly in their (2013) paper examining the Texas electricity grid. They chose the Texas grid since it had the largest penetration of intermittent renewable generation in the U.S., standing at 8% in their 2011 data. The latest data for 2015 indicates the Texas grid still leads the U.S., now at 12% with the western grid second at 8%.2
In spite of relatively low penetration of intermittent renewables, relative to ambitious goals for the future, the Texas grid is already experiencing large swings in wholesale electricity prices (see figure). Overnight lows in electricity demand drive down prices, since the wind tends to keep on coming at night, even as demand falls. Prices have even occasionally even gone negative on the Texas grid at night (meaning the grid would have been willing to directly pay a storage company to take the energy!).3
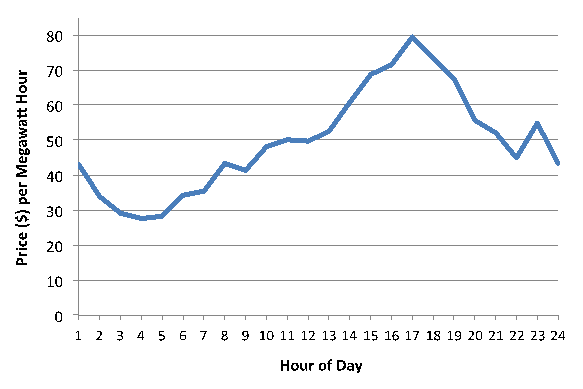
(data from Carson and Novan, 2013)
In a basic economic sense, the value of storing cheap (or even free) energy from low demand periods overnight and selling it during the afternoon could be calculated simply as the market price difference between the time periods.4 Instead of running more, expensive natural gas turbines during an afternoon demand peak, very cheap generation from the previous night could be discharged from a battery. However, such an analysis would be incomplete: changes in damage from pollution, for example through climate change or local health problems, will also occur and must be factored in.5 Carson and Novan perform this analysis, comparing pollution with and without the charging and discharging of added storage capability.
They find that while some of the effects of storage create clear environmental gains (for example wind farms do not need to be curtailed at night since the energy can be stored) other, simultaneous effects produce environmental losses. In particular, storage also makes it profitable to keep coal-fired power plants running at night. The net effect of added storage is a small increase in the total amount of pollution from the grid.6
A closely related paper (Holland and Mansur, 2008) does not look at battery storage, but at one of its competitors: “real-time pricing.” The idea is that instead of storing up extra generation to meet mismatched consumer demand, perhaps we could reshape demand to better match generation. By presenting consumers with lower prices at some times and higher prices at others it is possible to shift demand around, for example consumers could do laundry or charge an electric car when electricity prices are low. The authors consider the environmental impacts of such a system and find that, as with the case of storage, overall pollution increases slightly.7
These papers suggest that battery-storage, as well as other methods to more closely match supply and demand, could paradoxically increase rather than reduce pollution. This finding is most easily understood by considering the effects of storage on the fossil part of the grid: coal-fired electricity plants typically ramp up and down very slowly, making them similar to renewable energy in the sense that neither source of power is particularly agile in meeting changes in demand. Mandating or subsidizing storage on the grid helps all unresponsive and slow-moving power sources, including renewable power and coal power.
I think the key for policy going forward is to realize that this pattern of effects, drawn from data during the 00s, will most likely begin to change as the grid transitions away from fossil fuel. Consider for example a grid where all the coal-fired power plants have been closed down. In that world the remaining least responsive sources of electricity would be wind, solar, and sources like nuclear and certain natural gas and hydro facilities. Policy to put more storage on the grid in this world would no longer have the side-effect of helping coal, and is therefore much more likely to produce a net positive for the environment.
Other Benefits of Batteries
I have so far focused on the ability of batteries to move power across times of the day to more closely match cycles in renewables generation, but there are a host of other important benefits to the grid. Consider storm-related damage, for example. A large battery situated between a damaged transmission line and end-users could prevent a blackout. Similarly, stressed transmission networks could be relieved if battery storage close to end users could handle peaks in demand instead of further straining the transmission line.8
Very rapid charge and discharge cycles in the “frequency regulation” market are another high-value use, of particular importance in the PJM regional transmission market.9 Largely in response to this value of frequency regulation, PJM added 160 MW of new battery capacity in 2015 and became the top market in the U.S. for battery storage.10
Smaller scale batteries placed in individual homes and businesses (so-called “behind the meter” installations) also have considerable promise in mediating load swings and offering benefits to their owners during storms or transmission failures. Germany has one of the largest fleets of household-scale batteries, with over 20,000 residential batteries installed in 2015 alone.11 The potential to coordinate the behavior of many thousands of home-scale batteries at once offers even more exciting possibilities for the future, again creating the ability to move bulk quantities of renewable power from times of the day when it is generated to times when it is most valuable in demand.
Policy: Where to From Here?
One of the most important lessons from the environmental economics literature is that flexible incentives, such as environmental fees or tradable permits, typically offer lower efficiency cost (or greater benefits at the same cost) when compared to targeted mandates.12 This effect comes from a combination of factors, but I think the most important in this setting is technological uncertainty: It is possible that batteries will emerge as the cheapest and best way to move renewable generation across time, and so mandating them early on could accelerate the technological gains even further. However, if in retrospect a different technology proves superior, then mandating batteries may slow down the eventual adoption of the alternative technology.
Correcting for this uncertainty requires added flexibility in policy: for example consider the California mandate to add 1,325 megawatts of storage by 2020.13 Regulators could have implemented this with subsidies to hand-picked technologies, but they chose instead to offer flexible incentives per unit of storage no matter what the technology. Batteries of all sorts and methods as diverse as electric trains moving up (down) an incline to store (release) power are now under construction in the state. The hope is that a flexible policy will reward the best storage mechanism the most.
Zooming out even farther, though, consider the possibility that none of the various forms of storage prove to be cost-effective. Instead, perhaps the penetration of internet-enabled “smart” home appliances, thermostats, and electric cars will grow faster than expected. The process of matching supply and demand, with near-instant response, could instead be accomplished by cycling whole cities of air-conditioners and car-charging stations on and off as renewable generation cycles up and down.
In order to create the broadest policy incentive, one that could lead to a whole range of storage technologies, demand-side approaches like the one above, and other solutions not even thought of, we have to look to the heart of the problem: the pollution damage itself. An electricity grid where polluting sources pay for the damage they do to the environment would automatically bring in renewables. Renewables would in turn mean less agile power generation and the potential for large swings in electricity prices. Anticipated price swings would encourage a whole suite of storage and demand-shifting technologies. In effect, the low price of renewable power contrasted with the high price of fossil power would reward any utility (and the innovators and entrepreneurs the utility works with) that finds a way to use more of one and less of the other.
Absent the ability to charge fossil generators a fee for the health damage they cause local residents, or for the global implications they create for climate, we must turn to other policy alternatives. The best of these will create flexible incentives that mimic what would happen if fossil power were costlier to generate. A new storage facility helping a fossil fuel generator make more profits, for example, should not be given the same reward as one that helps a wind farm keep the turbines running all night. Similarly, batteries that enable higher profits or utilization rates for solar power should be encouraged.14 The challenge will be in differentiating the role of any given storage unit, perhaps by working to target policy toward storage that locates, or operates at times of day, most beneficial to renewables.
Finally, I think there is also a strong case to be made for subsidies to basic research in storage. While some innovations may only be possible through large-scale adoption, there are also considerable positive spillovers from fundamental innovation. If the bulk of electricity generation is to swing toward intermittent renewables, we must be ready with effective, affordable solutions that can match supply and demand.
Special thanks to the Kleinman Center for Energy Policy for hosting my visit, and to Dillon Weber for excellent research assistance.
Mark Jacobsen
Professor, University of California San DiegoMark Jacobsen is a professor of economics at the University of California, San Diego and a research associate at the National Bureau of Economic Research. In 2015-2016, he was a visiting scholar at the Kleinman Center.
Feature Image: Xcel Energy
Carson, Richard and Kevin Novan. 2013. “The Private and Social Economics of Bulk Electricity Storage.” Journal of Environmental Economics and Management
Cropper, Maureen and Wallace Oates. 1992. “Environmental Economics: A Survey.” Journal of Economic Literature 30, 675-740.
ERCOT. 2015 State of the Grid. Available at http://www.ercot.com/content/news/presentations/2016/2015_StateoftheGrid…
Fitzgerald, Garrett, James Mandel, Jesse Morris and Hervé Touati. 2015. The Economics of Battery Energy Storage: How multi-use, customer-sited batteries deliver the most services and value to customers and the grid.Rocky Mountain Institute, September 2015. Available at http://www.rmi.org/Content/Files/RMI-TheEconomicsOfBatteryEnergyStorage-….
GreenTech Media. 2016.US Energy Storage Market Grew 243% in 2015. March 03. 2016. Available at http://www.greentechmedia.com/articles/read/us-energy-storage-market-gre….
Holland, Stephen and Erin Mansur. 2008. “Is Real-Time Pricing Green? The Environmental Impacts of Electricity Demand Variance.” Review of Economics and Statistics 90 (3), 550–561.
J.P. Morgan Inc. 2015. Eye on the Market Annual Energy Paper: Deep De-Carbonization Of Electricity Grids. October, 2015. Available at https://www.jpmorgan.com/jpmpdf/1320687247153.pdf.
Mnyshenko, Oleksiy. 2015. Essays in Energy and Innovation. Doctoral dissertation, University of California, San Diego.
PJM Regional Transmission Market. 2016. PJM Fact Sheet: Electricity Storage. Available at https://www.pjm.com/~/media/about-pjm/newsroom/fact-sheets/electricity-s….
PV Magazine. 2016. “Solar Storage Outsells Electric Vehicles.” Available at http://www.pv-magazine.com/news/details/beitrag/germany–solarstorage-ou….
The Brattle Group. 2014. The Value of Distributed Electricity Storage in Texas. November 2014. Available at http://www.brattle.com/system/news/pdfs/000/000/749/original/The_Value_o….
WECC. 2015 State of the Interconnection. Available at https://www.wecc.biz/Reliability/2015%20SOTI%20Final.pdf
- See J.P. Morgan (2015) for a discussion of options to accomplish “deep de-carbonization.” [↩]
- Data for the Texas grid is from ERCOT (2015); most of the 12% is wind. Western grid data is from WECC (2015), where the 8% is a mix of wind and solar. [↩]
- This is the result of broader subsidies to wind generation, even at times of day when the grid would prefer generation be stopped. [↩]
- For a technical analysis of this value and a depiction of forecasting ability (for example how effective we can expect to be in charging and discharging batteries at the optimal time) see chapters two and three of Mnyshenko (2015). [↩]
- Note that in an economically efficient setting, where generators would be paying environmental taxes equal to the damage they cause, the simple analysis using the peak versus off-peak price difference would again be relevant. [↩]
- Carson and Novan (2013) find an increase in CO2 emissions of 0.19 tons for each additional megawatt-hour of electricity stored. [↩]
- For example, they show that a 10% reduction in the coefficient of variation within day (making demand smoother within each day) increases SO2 emissions by 0.4% and increases CO2 emissions by 0.2%. Cross-day smoothing produces similar, small increases in pollution. [↩]
- See Fitzgerald et al (2015) for a detailed discussion of these and other benefits battery storage can provide on the grid. [↩]
- See PJM (2016) fact sheet on storage. [↩]
- GreenTech Media (2016). [↩]
- PV Magazine (2016). [↩]
- For a readily accessible overview of this literature see Cropper and Oates (1992). [↩]
- See California Public Utilities Commission rulemaking 10-12-007. [↩]
- Solar, peaking at mid-day, could be slightly better matched to demand than wind (for example consider air conditioning) but storage could still increase the value of solar by smoothing high frequency peaks and valleys or moving the energy into the early evening. [↩]