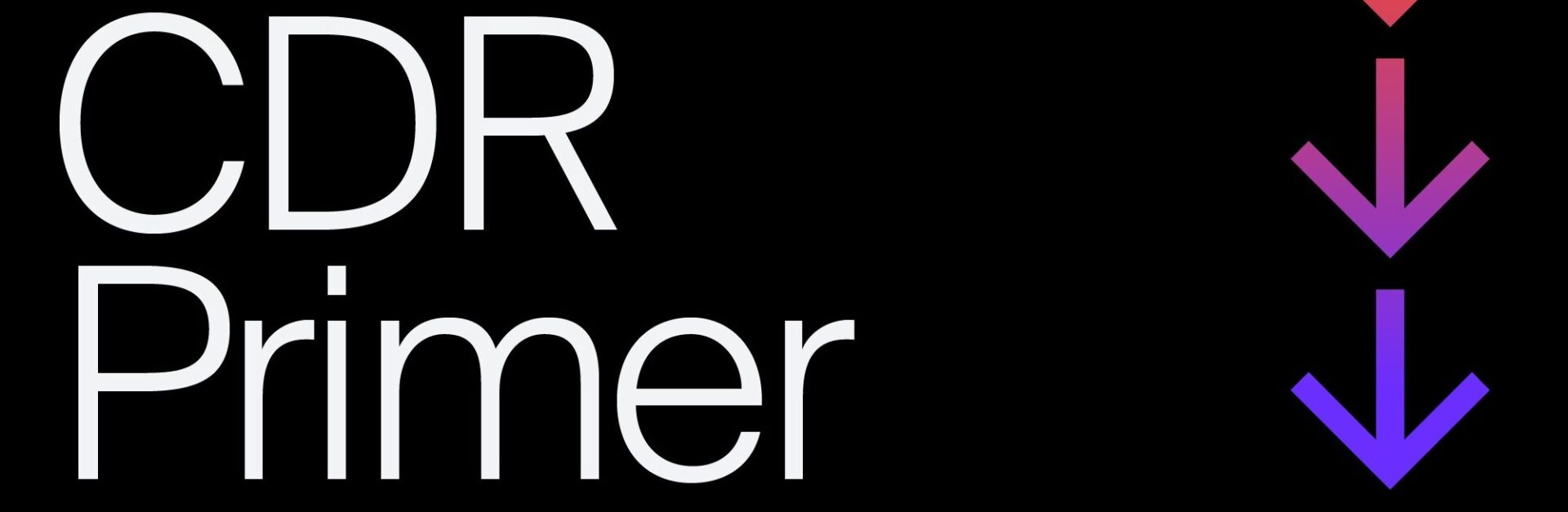
A Primer on Carbon Dioxide Removal
Carbon Dioxide Removal is an industrial-scale strategy to hold climate change in check. Five experts weigh in on CDR’s potential, challenges and moral hazards.
The global effort to slow the pace of climate change will require that two basic strategies be implemented on a massive scale. The first strategy is well known, and involves shifting away from today’s fossil-fuel dependent energy system, and toward a future where nearly everything will run on electricity produced by zero-carbon resources.
The second part of the effort to combat climate change has, until recently, attracted relatively less attention. Carbon dioxide removal is the process of removing carbon dioxide from Earth’s atmosphere. CDR can be used to offset some of today’s CO2 emissions, and might some day even be able to turn back the clock, by lowering the concentration of atmospheric carbon to levels that existed on an earlier, less hot Earth. CDR will be a key part of any plan to reach net-zero carbon emissions by the middle of this century, as the United States, the European Union, and a growing number of countries have proposed to do.
In the podcast, five experts discuss CDR in its many forms, from cutting edge technologies to fundamental nature-based processes, and explore the complex, industrial-scale undertaking that will be required to remove CO2 at scale. The guests, whose research is available in the newly published, online CDR Primer, also look at potential moral hazards, equity challenges and unforeseen consequences of carbon dioxide removal.
Andy Stone: Welcome to the Energy Policy Now podcast from the Kleinman Center for Energy Policy at the University of Pennsylvania. I’m Andy Stone. Slowing the pace of climate change will require two simultaneous efforts on a global scale. The first of these efforts is well known and involves shifting away from today’s fossil fuel-dependent energy system and toward a future where nearly everything will run on electricity produced by zero-carbon resources.
The second part of the effort to combat climate change has, until recently, attracted relatively less attention. Carbon dioxide removal is the process of removing carbon dioxide from Earth’s atmosphere. CDR, for short, can be used to offset some of today’s CO2 emissions and might someday even be able to turn back the clock by lowering the concentration of atmospheric carbon to levels that existed on an earlier, less hot Earth. CDR will be a key part of any plan to reach net-zero carbon emissions by the middle of this century, as the United States, the European Union, and a growing number of countries have proposed to do.
But what exactly is CDR? The answer is complex, as it is a term that encompasses everything from cutting-edge technologies to the most fundamental of nature-based processes. What is clear is the fact that implementing CDR at meaningful scale will be a complex, industrial-sized undertaking that is already raising weighty political, economic, and even moral questions. On today’s podcast, we’ll be taking a look at CDR with the help of a group of researchers who are behind the new CDR Primer, which is an online resource that covers many of the questions around CDR, from technologies to land use to economic and equity issues. Each of today’s five guests will offer highlights and takeaways from one chapter of the Primer. So without further ado, let’s go around for some introductions. Toly Rinberg, let’s start with you.
Toly Rinberg: Thanks for having me. My name is Toly Rinberg. I’m a Ph.D. student at Harvard University. I am working on a new principle for direct air capture that actually makes use of desalination technologies, but I also spend a lot of my time working on governance of carbon dioxide removal. Andrew Bergman and I co-authored Chapter 1, which lays out the case for CDR, bringing the science to the social justice framework.
Erica Belmont: I’m Erica Belmont. I’m an Associate Professor of Mechanical Engineering at the University of Wyoming. My expertise is in thermo-chemical conversion, particularly of solid feedstocks like biomass. My work includes pyrolysis, gasification, and combustion for the utilization of these feedstocks to generate energy in other useful products. Today I’ll be talking about Chapter 2 specifically of the Primer, which really covers the building blocks of CDR systems. These are the various CDR approaches that have been developed or are under development. Then specifically, I’ll be talking about biomass pathways to CDR, including biochar and biomass energy with carbon capture and sequestration, which is commonly called by its acronym BECCS.
Jeremy Freeman: I’m Jeremy Freeman. I’m the Executive Director of CarbonPlan. We are an organization focused on the scientific integrity and transparency of climate solutions. We’ve done a lot of work analyzing a wide range of carbon removal opportunities. CarbonPlan was involved in writing and editing the Primer. We also helped produce the Primer as a digital open-source online book, which so many of you are really excited to be involved in. Today I’ll be focused on Chapter 2, on the building blocks, and also Chapter 3 on mapping CDR opportunities.
Noah McQueen: Hi, I’m Noah McQueen, and I’m a Ph.D. student in Chemical Engineering here at the University of Pennsylvania. My specific expertise is in direct air capture, as well as carbon mineralization approaches to carbon dioxide removal. Today I’ll be talking a bit about direct air capture as a carbon removal approach, as well as about life cycle analysis and how it’s important in determining both the impact and net negativity of potential carbon dioxide removal systems.
Peter Psarras: I’m Pete Psarras. I’m a Research Professor at Penn, with a background in carbon capture, removal, and utilization. I also have done some work on life cycle analyses and the techno-economics of these technologies. I co-authored Chapters 4 and 5. I’ll be speaking specifically on Chapter 5, the role of utilization in carbon dioxide removal.
Stone: Thanks, guys. Before we jump into the chapters themselves, let’s do a little bit of an intro and a background on the Primer. First off, Toly — what is the need the CDR Primer is intended to fill?
Rinberg: If I can just set the stage, in the last few years, more than one hundred countries have announced that they’ll aim for what’s called a “net-zero emission target” by midcentury. Net-zero is an average, and what it really means is that countries are relying on the fact that they can account for all the emissions they do not eliminate by removing a proportional amount of CO2 directly from the atmosphere, so enough to compensate for any remaining emissions. The reason that this conversation is important is that although some amount of CDR is unavoidable, especially to offset what’s called “hard-to-avoid emissions,” there are also huge political and financial incentives to overstate the importance of CDR.
Gesturing at future scenarios that rely on large amounts of CDR is politically appealing because it avoids the societal burden of rapid and deep decarbonization that we urgently need today. In a real way, focusing on CDR really does decrease the chances of averting catastrophic climate change. We should be really clear who benefits from overstating the role of CDR. That’s the fossil fuel industry, a for-profit interest of fossil fuel executives and bankers who are working to sow confusion on this very point today. And we should also be very clear who stands to lose, and that’s the poorest and most vulnerable populations on this planet, and especially in the global South.
Unfortunately, the community of scientists and engineers working on CDR has also fed into this narrative by citing overstated CDR calamities as a reason to support and fund CDR research. So it is with this backdrop that our Primer aims to cut through the hype. I don’t know, maybe you hang out on Twitter and give folks, especially people who are just learning about the field for the first time, the right tools and language and framing to understand all these tricky and important concepts without throwing away paths that are too costly today — and by laying out a real social justice-based approach.
Stone: Pete, there is quite a backstory to the Primer. My understanding is that it began as a student project, and it pretty much grew from there. How did all this get off the ground?
Psarras: It all started in Calabria, Italy. Actually one of our former research associates in the Professor Wilcox group is Simona Liguori, a brilliant experimentalist, now with Clarkson University. So she hailed from Calabria, got us excited about the idea, and that was our first workshop. It was really just a group of experts discussing the state of carbon dioxide removal. This was a few years back. We were coming off of a huge wave of CCS, kind of in the mid-teens. We were starting to talk about things like utilization, but CDR particularly — this predated even the first commercial direct air capture plant. So CDR was really new.
What emerged from that meeting, outside of us all wanting to retire in Calabria, was that despite our common goals that were apparent, there was really no common language. A lot of that had to do with the fact that this was such a nascent field, but also because there are just a variety of approaches which lends to a lot of voices needed. So there is some recognition there to form a close-knit community around CDR. We rallied around that a bit, but we also realized that there could be an opportunity here to provide something to the greater community, as well.
So we followed that on with two more workshops, one in Edinburgh, and one in Worcester, Massachusetts. The Primer slowly took form. It was a student-led project, and we wanted it that way because this is a Primer. It’s for this new generation. We’re all-hands-on-deck, and we need as much help as we can get, but I think having students lead this effort made it a little bit more digestible, friendlier, easier on the eyes, so to speak. But experts were right there contributing, guiding, mentoring, and our collective conscience was born. Kudos to Jennifer Wilcox for really spearheading and organizing, but also to Ben Kolosz who is a Research Associate here at Penn, and of course Jeremy Freeman with CarbonPlan, who really helped steer this thing into form.
Stone: Jennifer Wilcox is a Professor here at the University of Pennsylvania’s Kleinman Center, who, as you mentioned, spearheaded this all with you. Is that right?
Psarras: Yes, it was her brainchild, and we were all happy to be part of it. She has a great way of rallying the troops, and we were collecting the minds around the world on this topic, again with the variety of approaches to pull us all together. That’s really what the Primer is all about.
Stone: It sounds to me like it’s really state-of-the-art on various aspects of CDR. It’s a guide for people to go forward, as well, right? What are the current levels of understanding, the development of the technologies, so everybody is on the same page, and so we know where we go from here? Is that a way to look at it?
Psarras: Yes, I think we tried to strike the balance between making it amenable to public consumption, not being too technical, but also educating in that sense, and educating a wide variety of audience, from policy-makers to early academics and the like, but also bringing to light a lot of the topics that Toly just mentioned — particularly a lot of the scale, the projections, and a lot of the EJ issues that are at least in a lot of people’s blind spots.
Stone: EJ being environmental justice.
Psarras: Yes.
Stone: As Toly said a couple of minutes ago — I just want to reference this. I read the Preface to the Primer, and it caught my attention. I had expected a discussion on technology, but really it was much broader than that, and it emphasizes that CDR is multidisciplinary. Pete, why is this the case?
Psarras: We’ve got to do so much in such a short time span, honestly. This is an all-hands-on-deck problem. I think you need the subject matter experts from soils, from understanding what’s going on in that first meter depth to the second, the effects on the ecosystems in oceans, to understanding mineralogy. We need engineers who understand how to scale. We need life cycle thinkers who understand how to assess the actual impact on climate, right? We need cost milers, to help develop low-cost, and how we can make these things economically viable. But we also need, as Toly mentioned, that EJ community, to ensure that we’re thinking about how this impacts people on the ground. We’ll get into it in a bit, but carbon dioxide removal — not CCS. This is not carbon capture and storage, where even though there are a variety of solutions there, they really all fall under the same umbrella.
We’ll term them broadly as “natural-based” versus “engineered.” But even that — that’s like calling the industrial sector the “industrial sector.” It does no justice to the amount of diversity in there. But all the same, these are all grounded by the same objective. We want to lower atmospheric stock of CO2, and we’ve wanted to do so for a long period of time.
Stone: So let’s move on to the first chapter, and that first chapter is titled “The Case for Carbon Dioxide Removal: From Science to Justice.” It starts out describing the challenge of addressing climate change. How does carbon dioxide removal fit into the larger response to climate change?
Rinberg: It’s good to start with the basics here. The accumulation of CO2 in the atmosphere as a result of fossil fuel combustion — land use changes is the main driver of the climate crisis. And CO2 is a very long-lived gas. It stays in the atmosphere for thousands of years, and it also mixes throughout our atmosphere quickly. So speaking strictly in terms of climate impacts, if we reduce a unit of CO2 emissions today, that’s equivalent to removing the same amount of CO2 from the atmosphere today. And so that sets up a trade-off. If we set a temperature target, then the less CO2 emissions are reduced, the more CDR we’ll have to do. But these options are actually not interchangeable, as that simple trade-off may suggest. To make this really concrete, if we for some reason decide to use CDR to compensate for the CO2 that we emit from burning fossil fuels, then we’re also allowing lead and particulate matter from those fossil fuels to continue to harm and kill people in frontline communities. And that isn’t being tracked, when we just consider the CO2 bouts.
So the societal and political and economic outcomes will be very different, depending on what emissions we decide to reduce versus how much we decide to remove. And I’ll just emphasize that. The least expensive option, if you just narrow things down to dollar per tonne, is not necessarily the best. If you take a broader perspective, that considers environmental justice and social justice broadly.
Stone: Let’s take a moment to clarify something here. What specifically does or does not qualify as carbon dioxide removal?
Rinberg: Yes, so there are a lot of concepts swirling around here, and it’s challenging because in some cases, the difference between carbon dioxide removal and avoiding emissions is more accurate to think about on a spectrum. Some processes are definitively carbon dioxide removal. Others really depend on the management and the way in which the system is structured. And I’ll emphasize that one challenge when labeling a process. A CDR system — and this is particularly challenging in biological systems like soil sequestration and reforestation — is that even though you can try to model the future, you may not know if a process removes more CO2 than it emits until years into the project. And there may be societal and physical forces that you’re not accounting for. So tools like life cycle analysis help with this. Noah will elaborate more when he covers Chapter 4 later on.
But what you should really consider is, and what is important for understanding these distinctions is where the CO2 is coming from and where it’s going. In terms of the input stream of CO2, to take one example, if you have CO2 that comes, say, from a natural gas power plant, you capture that CO2, and then you bake it into cement, let’s say. Then you’re storing that CO2 for a very long time, but what you’re doing there is just avoiding emissions. You’re preventing that CO2 that left that natural gas power plant from entering the atmosphere. That CO2 — you have to take CO2 from the atmosphere and put it into the cement in order for it to count as removal. What’s important here is that these might seem like simple concepts, but companies applying for CDR funding and companies funding CDR have created real confusion on this seemingly simple point. And that’s part of what the Primer attempts to address.
If I could also just say, I think another important concept that’s worth discussing is the idea of where the CO2 ends up and how it’s stored. So this is where the term “permanence” comes in, or how long carbon is kept outside of the atmosphere. Permanence helps us evaluate how a field of soil should be governed, which needs to be continuously managed, versus a direct air capture process, which if done properly, can basically store carbon effectively and indefinitely. So you can see how this gets very tricky very quickly.
A field of soil might technically be net-negative for the first five years, but then if management practices change, that carbon that has absorbed into the soil could fly right back up into the atmosphere, and then the whole process has been net-zero or maybe even a source of emissions. That’s not to say we shouldn’t pursue these soil options or other options, but we have to be really cognizant of the limitations. This affects regulations today. There are currently government-backed carbon offset soil protocols in California that are really not taking these permanent realities into account.
Stone: Interesting, so it’s not just about taking carbon dioxide out of the atmosphere. The key issue here is what happens to that carbon dioxide after that point. Is it stored permanently, or is it in some form that in the foreseeable future or much further down the road, it could be rereleased into the atmosphere? I think we’re going to get more into that, but that sounds like that’s a key, key issue here.
Rinberg: Exactly.
Stone: Now one other key point I wanted to bring up that you talk about in this first chapter is that CDR is going to have to be implemented on an absolutely huge scale to significantly reduce the concentration of carbon dioxide in the atmosphere. Can you put this scale into perspective?
Rinberg: Yes, this is such an important question, and it’s a point that a lot of folks are talking about — actually in a wrong way, and frankly in a dangerous way — that the Primer helps clarify. So how are people currently talking about the scale of CDR? Well, essentially conversations revolve around what are called “integrated assessment models,” which use economic, ecological, technological assumptions to model global emissions trajectories. And these scenarios are typically compared on the basis of cost. Again, that’s something like a dollar per tonne of CO2.
For example, scenarios keeping warming to 1.5 degrees Celsius typically have included CDR deployment between 5 and 15 billion tonnes of CO2 per year, around the year 2050. And there are some highly-cited CDR studies, such as the National Academies of Sciences’ Negative Emissions Technologies Report, which has a lot of fantastic resources. That report calls for a massive 20 billion tonnes of removal per year by the end of the century.
Actually, I have their quote. They actually frame this as a conclusion in the report. “If the goal for climate and economic growth are to be achieved, negative emission technologies will likely need to play a role in mitigating climate change by removing 10 gigatonnes per year of CO2 globally by midcentury, and 20 gigatonnes per year globally by the century’s end.” So for reference, we’re currently emitting about 40 billion tonnes of CO2 per year.
Stone: Forty billion a year.
Rinberg: That’s right. So that 20 billion per year would mean we’re sucking up 50% of current emissions. These are massive numbers.
Stone: Let me just stop you there for a moment. That is massive. So of all the emissions of all the automobiles, power plants, airplanes, whatever it may be, we’re talking about sucking half of that every year — half of the yearly emissions back every year, if I understand.
Rinberg: That’s correct, yes. And that’s what these reports are claiming, but I want to emphasize that in a lot of cases, what these reports are doing is framing this removal as necessary and required, and that language is actually not appropriate. What I mean by that is that by construction, these integrative assessment models are actually not evaluating what is required. They are evaluating the costs. Essentially they are making a cost analysis about picking the cheapest dollar-per-tonne option, given certain constraints. That’s not asking what are truly or almost unavoidable or very hard-to-avoid emissions that will be left, which is where we will need CDR to compensate for that.
And so calling these IM results necessary amounts of CDR unreasonably justifies potentially excessive and morally hazardous, really, amounts of CDR. So what Section 1.4 — what we present there is an alternative bottom-up approach about how to think about this. So we asked if the goal is to stop warming this century, how much CDR is truly required? CDR is only required to offset hard-to-avoid emissions, which we define as unacceptable to avoid from a social justice perspective, so like feeding humans or extremely physically difficult to eliminate within some time frame. And we didn’t ask about cost in this analysis at all. We assume we can decarbonize the entire electricity and industrial sectors, which is an extremely hard challenge. But even with these assumptions, we looked sector by sector, and we said, “What are these really hard-to-avoid emissions? Where will they be?”
And we found that most would come from agriculture and aviation, and taken together, the hard-to-avoid emissions would need between 1.5 and 3.1 billion tonnes of CO2, of CDR, to offset them. So to be clear, these are still very large numbers — 1.5 and 3.1 — but they’re also a lot smaller than what these integrative assessment models claim, between 10 to 20. Just as a reference, one billion tonnes of CO2 removal per year, if you run a direct air capture facility, will require approximately 10% of current global electricity production. Or you could grow a forest the size of Texas. So these numbers are still massive, but we should really understand what we’re doing when we’re pointing at these extremely large, 10-20 billion tonnes per year numbers, and the moral hazards associated with that.
Stone: So the needs are smaller, but the needs are still extremely large, as you point out. That gets to the next thing that you just also mentioned. You introduced in the chapter some of the potential moral hazards associated with CDR, which you just gave us a brief intro into. Can you give us more specifics about what hazards stand out?
Rinberg: Yes, one thing that I think stands out to me are just all the unknowns. It’s easy to focus on the carbon accounting, just as the net-zero concept suggests. All we have to do is balance the carbon budget, and we’re good. But there is so much, as Pete discussed earlier, as well, there is just so much that a CDR transformation would entail, in terms of changes in land use, in our energy system, in how we manage food and our industrial processes. We just have so little experience with such a massive societal transformation that’s needed to scale up CDR to key per tonne levels. And so if we aim for really large-scale CDR, we plan for that, and then we fail, then we’re hurting future generations, and those people aren’t able to consent to our decisions today.
Historically, when massive shifts have happened in society, lots of people tend to suffer. The only way we can really avoid that — or work to avoid that — is to embrace an equity-based approach, one that focuses on redistributing wealth and access to resources and privileges. We get at this in the last section of Chapter 1. From a personal perspective, this is why I think as important as it is to be careful about CDR and how we approach it, especially as it’s just getting a lot more attention these days, CDR is also this immense opportunity, if we approach it properly, to reduce inequality and prevent future harm.
If we’re going to embark on a massive CDR scale-up operation, democracy is key. The only way to really balance the enormous potential harms of climate change and the risks of possibly not scaling up the infrastructure is to really rethink how we collectively plan for and govern that infrastructure. And that includes challenging questions about how to move power away from polluting industries, but also considering options like public ownership of infrastructure. So we’ve got a lot of work ahead of us.
Stone: Erica, Toly and Pete pointed out that CDR is multidisciplinary. Now Chapter 2 looks at the full range of CDR systems. What are the general types of CDR, and why are so many approaches needed?
Belmont: Yes, CDR actually is multidisciplinary, and the Primer really covers a wide range of CDR approaches. These approaches are often generally classified as either nature-based or engineered solutions. Those are kind of broad categories under which we can classify these approaches. Nature-based solutions really focus on restoring or enhancing natural systems to uptake CO2 from the atmosphere and increase carbon storage, whereas engineered solutions, in contrast, are really technology-based. There are many different approaches described in the Primer, so I definitely recommend that listeners read the Primer for a really comprehensive list and descriptions. But I’ll just give some examples within these broad categories.
So nature-based solutions include approaches like forest management, aforestation, reforestation, soil carbon sequestration. Engineered solutions include approaches like direct air capture and biomass-based solutions like biochar and BECCS. Biochar and BECCS are often classified as engineered solutions, even though they utilize biomass or they have a nature-based component to them, but they still have the technology component, as well. And as you mentioned, there are many approaches, and that’s really a good thing, because each of these approaches has constraints that may limit its deployment potential. These constraints could include things like early technology maturity, questions of storage durability, costs, energy demands, and land use requirements. These challenges and limitations really suggest that we need a portfolio of CDR approaches to meet our CDR targets, and that’s really what the Primer sets out to describe.
Stone: I’d like to jump here for a moment to Noah. He has worked on direct air capture, which is arguably the most technology-focused of the CDR solutions, at least per my understanding. So Noah, what is direct air capture, and when and where is it a good solution?
McQueen: That’s a great question. Direct air capture or DAC, as you mentioned, is an engineered carbon removal approach. In its current iterations, companies use large fans to flow massive volumes of air through what are called “engineered contactors.” And these contactors contain a material that is meant to facilitate the contacts between this inlet air stream and to a given capture agent. This capture agent will selectively remove CO2 from that inlet air stream that allows for a “cleaner air stream” to exit the contactor.
So some versions of these air contactors use monoliths. You can envision them kind of like a catalytic converter that you see in your car. After that capture agent has removed enough CO2, it’s regenerated and can be used to capture more CO2. This can either take place in the contactor or downstream of the contactor, but it typically involves using either a higher temperature and/or a lower pressure condition. This regenerates the capture agent and then produces a high purity stream of CO2. So it’s important to note here that DAC is a process that produces a pure stream of CO2 and must be coupled to geologic storage to achieve negative emissions.
Focusing on the next part of your question of when and where is it a good solution, there are many resource considerations that affect where we should place these systems — which are outlined in detail in Chapter 3 of the Primer. But two of these resource considerations are the proximity to geologic storage and the energy resource availability. So since DAC produces a pure stream of CO2, you need to put it somewhere or locate it near transportation infrastructure, such as pipelines. And then DAC is also energy-intensive, which means that it’s important to co-locate direct air capture with low-carbon energy resources. So an optimal siting for direct air capture maximizes the proximity of the DAC system to the resource required for its operation.
Stone: Let me ask you a little bit more about that energy intensivity. Is that going to be a problem? That sounds like DAC could be competing for clean electricity to run the systems with general electricity demand.
McQueen: That’s a great question. Direct air capture is energy-intensive. A direct air capture plant requires between about 150 and 500 megawatts of energy to power the process, depending on the energy resource used. And this is equivalent to a small to mid-sized power plant, so you can imagine a direct air capture system coupled to a power plant. It’s important to say that we have to first focus on decarbonizing grid electricity production, and then expand the capacity to accommodate direct air capture. But unfortunately with our current climate situation, we’ve kind of moved into a period where we can’t really focus on one or the other but have to do them both in tandem.
Stone: So at the other end of the spectrum is forest management, which Jeremy has a lot of insight into? Jeremy, what role can forests play?
Freeman: That’s a great question, Andy. So forests and other vegetation more broadly achieve carbon removal through the chemical reactions of photosynthesis, with the carbon ultimately stored in the plant biomass. That’s happening all the time in our existing ecosystems, and there are lots of good reasons for preserving preserve forests, habitats, ecosystems, biodiversity. The role of forests specifically as a carbon removal strategy is a little more complicated. In that case, we’re usually talking about either enhancing the existing sync in a few different ways — and there’s a variety of ways to do that. One is reforestation, and that refers to restoring forests in existing previously forested areas. Aforestation refers to planting forests in new locations. And improved forest management refers to changing harvest practices so as to potentially increase the amount of carbon storage in the managed forest.
The basic mechanism of carbon removal here is relatively straight forward. I do want to stress there are some critical issues and some critical concerns. The first concern is that carbon removal is not permanent. There are many threats to forests. There’s fire, there’s drought, there are insects, and there is also, of course, the potential that the forest, while preserved for some period of time, ends up getting cut down. In the best case scenario, the carbon is maybe stored for a hundred years. In comparison, some of the methods like what Noah was talking about involving geological sequestration — those can last for thousands of years. I really want to stress this is important because the carbon dioxide emissions that we’re constantly producing — those have effects on the atmosphere and on global warming that last for hundreds to thousands of years. So we need to have carbon removal strategies that are kind of matched to that, and that’s a real challenge when it comes to systems involving forests.
The other really important thing to stress around forests, especially when we’re talking about those strategies that involve changes to existing land management practices, is that we really have to think hard about a thorny problem called “additionality.” The idea here is that if someone says they are not going to cut down a forest or they are going to harvest it less frequently because you paid them money to do it, for example, as is done in forest offset regimes, how do we really know what they were going to do in the first place? That has to be a component as to how we think about incentivizing and evaluating the efficacy of these methods. There’s a lot of good evidence that, at least in the past, programs like this haven’t really worked, and there have been a lot of problems.
So again, forests are fantastic for all kinds of reasons. Their mechanism for CDR is relatively straight forward, but there are a lot of concerns to be worried about, especially around permanence and around additionality — if you’re considering them specifically for carbon removal.
Stone: Direct air capture, as Noah was talking about a few minutes ago, seems to take relatively little space. It’s machinery, whereas forestry solutions are just the opposite. It takes so much land space. What challenges result from that need for land?
Freeman: Yes, great question, and we’re surely going to come back to this in the chapter on mapping, as well. I’ll say one thing. Actually, one of the first things Noah taught me about this whole space — direct air capture can take up a lot of space, too, especially because we’re often talking about building new energy resources alongside building our direct air capture resources. So there are space requirements there, as well. Forest and actually a lot of the bioenergy things that Erica works on — that can be even more expansive, and in particular you’re often talking there about using land that could well be used or desired for lots of other competing purposes, whether agriculture or places for humans to live. It’s incredibly complicated, and really all of these strategies, if you imagine projecting them out to massive scale, start to have a lot of complicated dependencies on land use that are really important to think through. They raise issues around governance and justice and local communities. I can’t stress enough how important it is to think about that.
Stone: Now Erica, you’ve looked at a solution that combines carbon capture and land management, in this case in agriculture. It’s called bioenergy with carbon capture and storage. How does this BECCS, which is the acronym, work? And in what circumstances is it a solution?
Belmont: Yes, so BECCS is a pathway to capturing carbon from the air and storing it in the ground while generating some useful energy products in the process. And so these energy products could be electricity, heat, hydrogen, liquid fuels — so really quite a suite of products and quite a variety of different pathways that are implied by that. And I’ll come back to that in a moment. But in terms of the carbon capture component, one of the rather unique things about BECCS is that it really combines a nature-based approach to taking the CO2 out of the atmosphere in the growth of the biomass itself, with the technology approach to converting that biomass to one of those useful energy products and then creating a form of carbon for storage and removal. So it’s a rather unique hybrid of nature-based and technology-based approaches in that way.
Now one of the interesting things about BECCS and one of the reasons it can be rather confusing to think about and summarize as people think about BECCS is that it’s really an umbrella under which many different biomass conversation pathways fall. And so these different pathways can look like combustion of biomass to produce electricity and heat, gasification to produce hydrogen and liquid fuels, pyrolysis to produce liquid fuels and/or biochar, fermentation to make fuels. And so through these different processes, the carbon in the biomass is converted to one or more of those different products I mentioned earlier, as well as some CO2 and/or biochar that are then the candidates for storage and removal. So some to all of the carbon is converted to a form that’s a candidate for removal.
As you can tell from that list of technology pathways, these are really very different, with very different energy products. And so from a CDR perspective, that means that there are also really different implications for these pathways. Number one, how much of the carbon in the biomass is available for storage and removal, and what the life cycle emissions are for each of those processes. And so again, under that BECCS umbrella, there are a lot of different considerations right there.
Another major consideration for BECCS is the biomass demand of this approach, and this is really both a major concern about BECCS and also a major limiting factor for BECCS. When we’re looking for biomass to use for BECCS, there’s a really important distinction to be made between biomass waste versus dedicated biomass. So if we think about using biomass waste, we’re thinking of using things like forest residues and agricultural residues with an eye towards things that wouldn’t be used for another purpose and would maybe be fated for decomposition or open burning, for example. Whereas dedicated biomass would include things like crops grown intentionally for BECCS, and that’s really where the agriculture component comes in, and the land use considerations and potential substantial land demand for BECCS comes in.
But among those biomass wastes is considered to be a safer pathway to BECCS because it doesn’t require land use repurposing for biomass production which, again, can have really serious adverse life cycle implications and other negative implications. But either way, whether we pursue waste biomass and/or some rollout of dedicated biomass for BECCS, we really are running into that scale potential effect. So there is a scale potential that has limitations, and really it reminds us why we need a portfolio of CDR solutions.
Stone: Erica, thanks very much. Let’s move on to Chapter 3 and Jeremy. So far we’ve talked about a number of CDR solutions, and one thing that’s really becoming clear is that each solution makes sense in certain locations. You’ve thought a lot about mapping CDR opportunities. What factors do you need to take into account when determining what CDR solution may work for a given location?
Freeman: Yes, so nearly all CDR methods have a fairly complex dependency on land and location. The key issues, what those factors are, vary a lot by category. So when it comes to forests and other biological systems, we need to think about available land. We need to think about how that land is currently used. Are there existing ecosystems or not? Was that land historically ever forested in the recent past or not? And if it has never been forested, why not? What are the ecological properties, climate properties of that location?
I will add that many ecologists really question the feasibility of converting unforested land to new forests, so it’s not clear that there are many opportunities for that, period. And some recent estimates, I also want to stress that the amount of potential new forest — reforestation or aforestation that we could do — have been wildly overstated. So it’s really important to be careful about what we’re assuming about available land.
A really important thing also with forests, and potentially new forests, is competition with agriculture, which very quickly becomes an issue. So those are some of the complexities with forests. When it comes to engineered systems like direct air capture, really key questions involve energy availability and then locations for mineralization or geological injection.
I want to call out Helene and Ben, co-authors on the chapter. They did some incredible work to basically systematically map low-carbon sources of energy like concentrated solar power, photovoltaic, and offshore wind. And then in parallel, they generated these beautiful maps of prospective sedimentary basins, and also maps of mafic and ultramafic rocks. And then what they did is look at how well these different maps co-occur, which can help suggest locations that it might be useful to explore doing these activities.
I’m using a lot of weasel words there because I really want to stress there are so many factors that need to be considered when it comes to citing and evaluating the potential for CDR deployment. And this chapter, Chapter 3, really just scratches the surface. There are some beautiful maps. There is some really elegant analysis and insight in there, but so much else needs to be considered. Local communities, regional governments, existing uses of land — it’s enormously complicated, and so many people and so many perspectives have to be part of that conversation and are critical to consider. So the data, the analysis, it’s exciting, and there’s a lot to learn from thinking about this mapping and doing this kind of mapping exercise, but there’s so much more to still consider.
Stone: What you’re saying really brings to mind the next question. There are so many considerations here, so many variables. So the question is, then, what kinds of datasets and statistical analyses are involved in doing this kind of mapping work?
Freeman: Yes, totally. I just love this kind of stuff. This was a fun, messy, super complicated data problem. Many of these datasets come from different sources in different formats and different resolutions — different levels of granularity. In some cases, we’re extracting numbers from PDFs. Some are images published as parts of papers in a wide variety of formats. It was really an enormous amount of work to get all these kinds of raw sources, get them into the same format, pull them in to do spatial analytics. Helene, Ben, and Grace who are also co-authors on the chapter, working on the forests and grass lines and soil side of things, to just bring all that together. They’re all heroes.
I also want to call out — this was a really exciting place in the Primer for our designers, Jonny Black and Richard Roche from Ordinary Things to really work hands-on with the team and figure out how to best visualize and display this information. With any problem like this, there’s the analysis, and then there’s also how do you look at it? Jonny and Richard just brought an incredible eye to the whole project, and I want to give them a lot of credit.
Stone: During our discussion of Chapter 2, I asked you a question about the land footprint of carbon sequestration with biological systems, particularly for example with bioenergy crops. I wanted to go a little bit deeper on that. That land footprint is massive. How should we think about those big land demands?
Freeman: It’s a great question, and it does, again, get really interesting and complicated in different ways for the different categories. I will always stress engineered systems also have potentially large land requirements. It depends on how we do them. In particular situations where we’re talking about constructing new low-carbon energy sources alongside direct air capture facilities, that’s going to result in a fairly large land requirement, as well. But certainly when we’re talking about aforestation, new forests, and when we’re talking in the context of BECCS — if we’re talking about, as Erica pointed out earlier, new crops, new growth — yes, the land demand gets big quickly.
I often think with this stuff, you’ve got to think at different levels of scale. The answer for what it would take to do a fairly small-scale pilot or a moderate-scale deployment is really different than what it would take to get to a gigatonne with one method. When you start getting to those kinds of frankly outlandish numbers, you start to really have serious questions about is this possible? What does it start to compete with? What questions do we need to be asking about other uses of that land, issues of local communities, issues of local governance, who is deciding what we’re doing? These are incredibly complicated questions and absolutely we need to be thinking about justice, and we need to be thinking about environmental integrity and ecosystems — all of that together.
I’d be reluctant to say there’s a hard limit on what’s possible for any of these things. Certainly at some point there will be bounds. I think that’s well outside what anyone thinks is possible right now, but we’re partly doing this work because we’re trying to prepare for the future, so we need to be thinking ahead to what it looks like to do these things that we’re maybe just now starting to consider. What does it look like to do them at a very large scale, and what are the consequences of that? We have to be really careful and really serious about that.
Stone: Jeremy, thanks. Let’s go on to Chapter 4 with Noah now. So Noah, your work looks at life cycle analysis or an understanding of the net benefits of a given carbon dioxide removal solution. Again, this is Chapter 4 we’re talking about. What does life cycle analysis take into account?
McQueen: That’s a great question. Life cycle analysis or LCA is an evaluation of what goes into, what comes out of, and the potential environmental impacts of a given system throughout its life cycle. So this can provide insight into things like what resources are needed to create or maintain the system, and this includes energy resources, materials, water, land use, among other types of resources. It really aids in understanding what parts of the process have the highest potential trade-offs or environmental impacts, which are also known as the processes’ hot spots. If you do this early on in the development of a process, you can really design specifically to mitigate the impact of some of these process hot spots.
So one specific aspect of life cycle analysis that is important for evaluating whether a system actually achieves net-negative emissions is carbon accounting. This involves quantifying the carbon emissions that will occur as a result of deploying a process, and it includes, to some people’s surprise, varying types of carbon emissions that need to be included for a comprehensive analysis. So I actually will think it’s beneficial to outline some of these and provide examples so that there’s a visualization of what types of emissions you can see from some of these carbon dioxide removal systems.
The first type of emissions is Scope 1, or called “direct process emissions,” and this refers to emissions that result directly from a given process. For example, if you have some kind of a chemical reaction occurring that produces CO2 that you then vent to the atmosphere, that’s considered process emissions. Similarly, if you have a kiln that combusts natural gas, where the CO2 from that combustion is also vented to the atmosphere, that constitutes direct process emissions.
The second type of CO2 emissions are called Scope 2 or “indirect emissions,” and these result indirectly from resources used by the process. For example, if your process uses grid electricity that is supplied by a natural gas combine cycle per se, the emissions from the electricity used in that process are considered indirect emissions. So your process requires 4 gigajoules of electricity; the emissions associated with producing those 4 gigajoules for your process would be considered indirect emissions.
And then the final type are Scope 3 emissions or “embodied emissions,” and this refers to emissions that result from the production or use of any good or the provision of any service, which sounds very complicated in a sense because it is. For example, if you have a reaction vessel that’s made of steel, or you use concrete to lay the foundation of a building, all of those materials have associated CO2 emissions that have to be factored into the overall footprint of the process. So when you think about these different types of emissions, you can imagine that what you choose to define as your processes also has a large impact on the results of a life cycle analysis. You can take DAC as an example of that. If you focus just on direct air capture, it will change the results of the life cycle analysis, as opposed to focusing on direct air capture coupled to, say, geologic storage. So to truly evaluate the impact of a proposed carbon dioxide removal approach, you need to consider the potential trade-offs and impacts across the entire system life cycle. This includes construction of the process, everything that goes into and comes out of the process, as well as the end-of-life considerations. So at the end, is the process demolished? Do you recycle any of that material? Can you recycle any of that material? All of these are things to consider when performing an LCA.
[OVERTALK] Including the complete life cycle of the system is known as the “cradle-to-grave life cycle,” and it’s really the only way you can determine whether a system truly achieves those net-negative emissions.
Stone: So what you’re saying is really, really important. It’s not just about the process itself. Everything that goes into even creating the equipment or whatever it may be for that process really has to be taken in account. So overall, on net, has this been carbon-neutral, positive, negative, whatever it may be? That sounds like it’s a really important takeaway here.
McQueen: Yes, absolutely.
Stone: A related question: Is the focus of LCA, life cycle analysis, therefore limited to carbon dioxide emissions, or can you quantify other aspects, as well?
McQueen:Â Oh, absolutely. You should definitely quantify other aspects, and life cycle analyses should take into consideration a myriad of different potential impact areas, and they typically do. There are a lot of common impact areas that are included in these types of analyses — for example, particulate matter. So all of the solid or liquid particles that are suspended in air, many of which are actually hazardous to people, you can quantify the particulate matter emissions from a given process, depending on the size of the matter that has evolved from the process. Similarly, there are impacts like eutrophication, which is an excess richness of nutrients, typically nitrogen or phosphorus in a body of water. These typically occur from runoff nitrogen, specifically from agricultural lands, and then phosphorus from common household detergents. And what this does is it causes a dense growth of plant life that depletes an area of oxygen and can cause death to animals. So you can quantify the expected eutrophication from a process.
Similarly, there are aspects like ecotoxicity, or how toxic compounds are to the environment — as well as human toxicity, which is the same as ecotoxicity but for humans. These potential impact areas can help us understand how the process impacts its environment, as well as what the trade-offs of the process are. So if one process has higher CO2 emissions but lower human toxicity, and another process has higher human toxicity but lower CO2 emissions, you have to consider all of those facets of impacts when deciding which system you’re going to pursue.
All of that being said, there are some areas that LCA does not do well in addressing, and this includes aspects such as societal or sociological impacts of a given process. This has given rise to a new field of LCA called social life cycle analysis, which really attempts to quantify the social impact of product systems throughout their life cycle. This includes things like impacts on stakeholders, like workers or local communities, in a range of different impact categories, including human rights and cultural heritage, among others. However, quantifying qualitative social impacts can pose a challenge to these types of life cycle analyses.
Stone: The range of factors that you have to take into consideration and the range of data inputs that would go into any modeling seem absolutely astounding and really, really large. So what challenges are there to getting the data to build the impact models?
McQueen: Many challenges. The first, and perhaps one of the most important challenges, is the data availability and accuracy. As you mentioned, to build these LCA models, you need tons of data, and you need a very good understanding of the process that you’re evaluating, which does require extensive amounts of data sometimes associated with individual components of these processes. So this builds the basic foundation for the life cycle analysis.
So if there are inaccuracies in the data used to perform the LCA, it kind of jeopardizes that foundation. One way that researchers would go about combating this is by coupling the life cycle analysis to an uncertainty analysis, which helps quantify the deviations in the data or account for any uncertainties that you see in data availability or accuracy over the literature. Data availability is a significant issue for developing technologies, which actually includes a lot of these carbon dioxide removal approaches. So since many of these systems have yet to be developed, there is increased uncertainty surrounding the data used to create these life cycle analyses — or you have to use similar process units as a baseline in determining what those potential impacts might look like.
Stone: Okay, let’s move on to Chapter 5, and Pete is coming back to talk about that one. So Pete, as mentioned earlier, carbon dioxide doesn’t necessarily need to be buried underground to take us on the path to net-zero emissions. Carbon dioxide can also be used in a variety of products, which is known as “carbon utilization.” My understanding is that this might actually improve the economics of CDR. But can you give us an introduction into carbon utilization, again, which is the focus of Chapter 5?
Psarras: Sure, carbon utilization in the simplest sense is converting CO2 to value. So CO2 is traditionally thought of as a waste product, right? There is usually no economic value to a waste product. We are in the precipice of collecting a whole lot of it, and so you’ve got to think of the problem. We’ve thought of capturing and removing a tremendous amount of carbon dioxide and what to do with it. Well, carbon is also a tremendous building block. If you were to look around your room right now, your home, just about everything you see from the clothes on your back to the roof over your head is built from carbon. We’re talking plastics, chemicals, fuels, wood products, concrete, the soles of your shoes — all these things could be made from carbon. So we’re really staring down, I think, a tremendous opportunity to generate revenue and wealth. And again, why would we want to do that? Again, we can improve the economics of carbon dioxide removal. It’s an expensive prospect, and traditionally feedstocks aren’t free, so people will pay you for this carbon dioxide, if you can find the right partnerships. You can think about this as sort of a mining opportunity, right? We’re taking carbon dioxide and turning it into a marketable commodity.
There are other benefits here. The majority of carbon in our commodities today comes from fossil. So I think the more carbon dioxide we can use in place, the quicker we can transition away from fossil. And as mentioned earlier, there are opportunities for local storage and long-lived products.
Stone: So this would be utilized primarily as kind of a synthetic fossil fuel? Is that what we’re talking about?
Psarras: That’s one option, right? There is a host — really too many to talk through when you think about taking carbon as a building block. Hydrocarbons, fuels are certainly one opportunity, and so the opportunity to displace fossil as a fuel is one, but I think a lot of fossil ends up in other products that we’re not quite as aware of when we talk about trying to defossilize and shift away. Part of that is finding replacements for those feedstocks, and carbon dioxide can be one.
Stone: So as you also point out in the chapter, there is debate as to whether CO2 should in fact be utilized at all. What are some of the concerns around carbon dioxide utilization?
Psarras: Right, we have to be careful. Just because you can use carbon dioxide, for example in a process to replace a conventional or incumbent route, you’d better be very certain that you’re not generating more carbon dioxide in the process, right? More CO2 is actually emitted to the atmosphere than had you done nothing at all, right?
And that’s where, I think, the life cycle analysis comes in. That’s a lot of what Noah talked about in Chapter 4, why that’s so important to use that to identify those processes that lead to net reductions from net removal. I hope by this point in the podcast that listeners understand that those terms aren’t at all interchangeable.
I think there is also great concern, as Toly pointed to in Chapter 1, where some of the prominent players in utilization, namely the oil and gas industry, have historically catered to their bottom line. So the motivation there may be unclear, and people have the right to be skeptical. But if we take a step back from those concerns, there has been opposition to utilization for quite some time in a very general sense. There is also opposition as it pertains specifically to CDR. I think the CDR viewpoints are a little more straight forward. It can change the outcome. If the name of the game is to remove carbon permanently from the atmosphere or over relative time scales, utilization is the back end of that process now. Instead of storage, we remove storage. We can completely change the course of that. It’s almost like vacuuming a carpet, but you don’t have a bag on that vacuum. You’re just really pushing things around the carpet. You’re not cleaning up, right?
Take the first direct air capture plant, Climeworks. So you’re removing carbon dioxide from the atmosphere. You pass your pipeline to an adjacent greenhouse. You boost crop yields, and then you vent that carbon dioxide. Where are you venting? You’re venting back to the atmosphere, and so this is cyclical. You can stand back, and the opponents will say, “How much CO2 did you actually remove in that process?” The supporters will say, “That direct air capture plant changed the world,” and it really launched a movement here that I think really gave birth to a lot of the things we’re talking about today — and a lot of energy to it.
From a more general sense, utilization has been viewed — and this goes back to CCS, right? — as a costly distraction to permanent storage. The argument is traditionally, why not put it underground? Just put it underground, let it be. Remove it permanently and securely for a long period of time. And it’s extremely hard to argue with that argument in a vacuum. But I find the logic just a little bit flawed. First, I think it assumes that the CO2 is already captured. It assumes that the capturing and removing technology was already in place and would have existed — and this is important — in the absence of a utilization partner.
So I think that argument ignores often challenging economics of capture and removal and really the power of utilization and one of the benefits to help subsidize those costs. [OVERTALK]
An additional point, I think, and this is also important and more on our radar now — I think it ignores some of the current challenges in saline storage. I think we understand storage. We know it’s the ultimate end goal, but this isn’t just about money. This is about permitting. This is about public acceptance, some geopolitical barriers. I think storage is a little bit more complicated than it should be at this point in our effort and the urgency that we have at hand. I don’t think we have the time to wait. And I think one of the fears cast by opposition groups is that we’ll open some type of utilization rabbit hole, leading us into technical lock-in that will actually make it harder to transition to storage when it’s ready, and when that infrastructure is in place. In the end, that could end up being more harmful and counterproductive to our goals than any of these technical and cost learning that we’d unlock through CO2 utilization as a means to help deploy some of these technologies in the interim.
Stone: It’s kind of analogous to the debate we’re seeing right now about natural gas infrastructure, right? Do you build the pipelines and then lock in and need to use those pipelines, and then obviously the use of gas, the issue of gas going forward. You’re talking about the same kind of thing with this potential utilization path. Is that correct?
Psarras: Yes. I think that’s a good analogy there, to typically lock-in it, you kind of reach a tipping point where it becomes kind of economically prohibitive to abandon those pathways. But I think there are mechanisms that we can look into to avoid that, and I think there are enough people who are actually thinking through these concerns to develop some kinds of strategic pathways around them.
Stone: Could policy make carbon utilization more relevant? Are there any policy solutions to grow this?
Psarras: I think it certainly can. There are certain economic incentives for utilization. We can see those today, our R&D support. We can get all the way to procurement and mandates in terms of supporting and shifting market spaces towards some of these lower-carbon commodities which will obviously be yielded, hopefully. I think that we talked through those life cycle issues that will be kind of a product of this. I think this comes back — not that there are mechanisms that can be helpful. The real question is should we do that? And really, I would say there are two schools of thought on that, and there are really two camps. And that may be oversimplifying, but really there are those who believe that utilization is an essential part of supporting early tech, promoting scaling efforts, driving down costs, establishing markets and infrastructure. And it will really set us in a position to succeed on our path to achieve those very lofty, kind of midcentury goals that Toly had outlined. And then there are those who feel that utilization could perhaps derail those efforts and hinder our pace relative to a counterfactual, were we not to pursue those avenues.
I tend to fall more in the first camp. I do think policy has a role to play in ensuring that that second reality is not realized. And I think there are a lot of conversations today surrounding that. Perhaps that means amending incentives so that there is a progressive gap between saline storage and use over time, or standardizing emission accounting schemes to thwart private interest groups and corporate greenwashing.
I think the will is there. I think we’re all watching. And really, back to what the Primer is all about, the more we educate the public on this, the more we cast light on these issues, I think the less likely we’ll head down a path of no return.
Stone: Erica, Toly, Jeremy, Noah, and Pete — thanks for talking.
Belmont: Thanks for having me. It’s great to be here with everyone.
Freeman: Thanks for having us, Andy.
Psarras: Thanks so much, Andy. I really appreciate it.
Rinberg: Thanks, Andy.
McQueen: Yes, thank you, Andy. It was great to be here.
Stone: For a deeper dive into carbon dioxide removal, visit the Carbon Dioxide Primer website at CDRPrimer.org. And for news and insights into a wealth of energy and environmental policy issues, visit the Kleinman Center website. There you can even sign up to get our monthly email newsletter, with updates on Center research, publications, events, and even this podcast. Thanks for listening to Energy Policy Now, and have a great day.
Erica Belmont
Assistant Professor, University of WyomingErica L. Belmont is Assistant Professor of Mechanical Engineering at the University of Wyoming. She oversees the Belmont Energy Research Group.
Jeremy Freeman
Executive Director, CarbonPlanJeremy Freeman is the Founder and Executive Director at CarbonPlan.
Noah McQueen
Doctoral StudentNoah McQueen is a Ph.D. student in Chemical Engineering at the University of Pennsylvania.
Peter Psarras
Research Assistant Professor of Chemical EngineeringPeter Psarras is a research assistant professor in chemical and biomolecular engineering at the School of Engineering and Applied Sciences and the Kleinman Center for Energy Policy.
Toly Rinberg
Doctoral Student, Harvard UniversityToly Rinberg is an Applied Physics Ph.D. Student at Harvard University.
Andy Stone
Energy Policy Now Host and ProducerAndy Stone is producer and host of Energy Policy Now, the Kleinman Center’s podcast series. He previously worked in business planning with PJM Interconnection and was a senior energy reporter at Forbes Magazine.