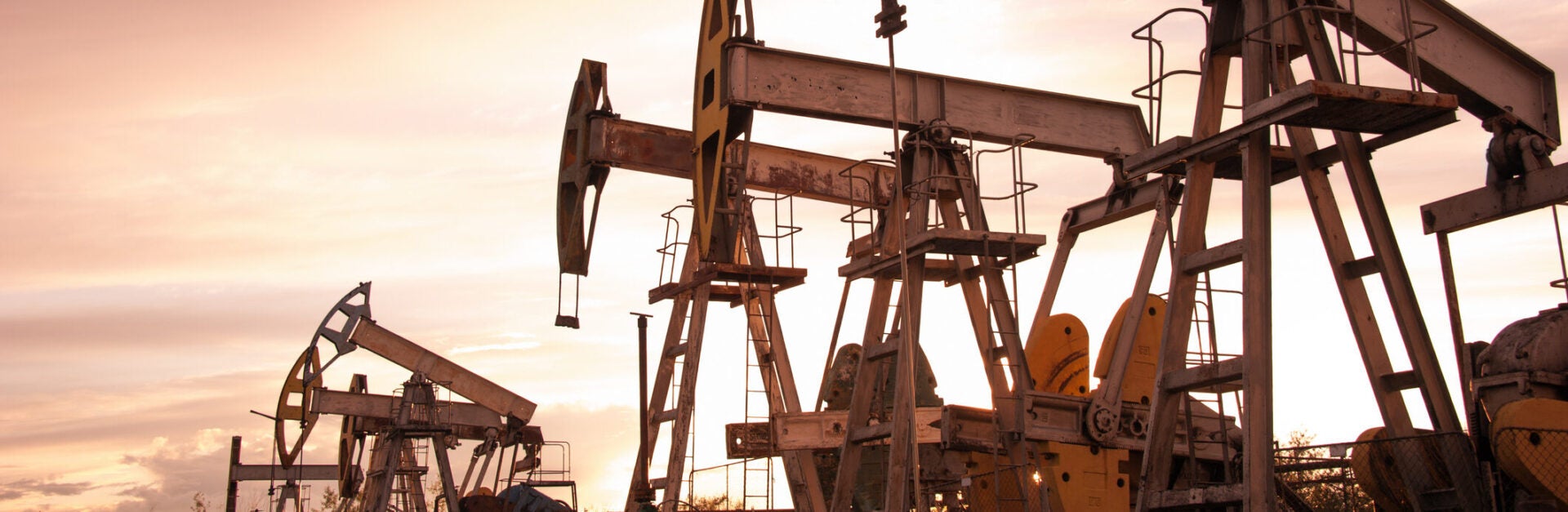
Exploring Direct Air Capture’s Role in Enhanced Oil Recovery
A type of carbon dioxide removal known as direct air capture, or DAC, relies on machines to pull carbon dioxide from the air. This technology, not yet built to scale, is finding a home in enhanced oil recovery. Here's why.
Earlier this year, the Intergovernmental Panel on Climate Change identified carbon dioxide removal as an essential tool in the global effort to achieve net-zero carbon emissions. One type of carbon dioxide removal known as direct air capture, or DAC, relies on machines to pull carbon dioxide from the air. This technology is not yet built to scale.
A wave of recent investment commitments brings hope development will accelerate. The commitments include $3.5 billion in the Infrastructure Investment and Jobs Act and over $1 billion from the fossil fuel industry to support direct air capture projects that use captured carbon dioxide to increase oil production at existing wells. This process is known as enhanced oil recovery (EOR).
To learn more, we spoke with Peter Psarras, a research assistant professor of chemical engineering at Penn, and head of the Clean Energy Conversions Lab, an affiliated lab of the Kleinman Center.
Q: What is DAC and how is it different from other carbon capture approaches?
A: Direct air capture is an engineered carbon dioxide removal solution. In the last decade, you’ve heard more about carbon capture and storage, or CCS. The key difference is that CCS is aimed at point sources, like power facilities and industry, including steel and cement producers that are notoriously carbon intensive. DAC, on the other hand, is one form of carbon dioxide removal (CDR) that targets CO2 that’s already been released—it’s like a time machine that makes up for decades of inaction on GHG emissions:
Q: What is enhanced oil recovery and why connect it with DAC?
A: Once we capture CO2 from the air with DAC, we need a place to put it. This is where enhanced oil recovery may be part of the answer.
Enhanced oil recovery squeezes the last bits of oil out of an oil field—producing oil that could not be recovered under conventional means. There are many approaches, but one called CO2-EOR uses carbon dioxide—injecting it deep into the earth to extract oil.
Already CO2-EOR is the largest user of carbon dioxide in this country—between 60 and 70 million tons of carbon dioxide per year is injected and stored in the subsurface. But most of this CO2 is sourced from natural underground reservoirs, meaning that the stored CO2 has no real climate benefit. Further, the produced oil will release CO2 back to the atmosphere upon combustion, so the net effect isn’t all that different from conventional fuel: carbon from underground ends up in the atmosphere. Pairing EOR with direct air capture is slightly different in that instead of adding new CO2 to the atmosphere, you’re cycling CO2 back and forth from the atmosphere to the technosphere.
Q: There is a lot of controversy surrounding the combination of direct air capture and enhanced oil recovery. Why?
A: Well, let me step back and say that direct air capture in and of itself is controversial, because it can be seen as a safety net that might limit our reduction actions today or enable “business-as-usual” practices.
The truth is we need to reduce emissions as aggressively as possible. Where we can’t reduce, we must capture from hard-to-abate industries first (like the transportation sector or aviation). Then—and only then—we can turn to DAC. The problem is that because we need to remove so much carbon dioxide (a gigaton per year by midcentury) that we have to talk about DAC today.
As for EOR, well, it’s a mechanism to produce fossil energy, which makes it controversial, too. We are supposed to be sprinting in the absolute opposite direction, away from fossil fuels, right?
The point is, we need to figure out how to do both simultaneously: aggressively cut emissions but also begin scaling DAC and other CDR now—so we’re not behind when we need it most.
Pairing DAC with EOR is a way to scale DAC today. EOR can be an economic driver for the development of direct air capture because it offers a stable source of revenue. When you look at the players in carbon capture and storage globally, 22 of 27 projects right now are tied to enhanced oil recovery. CCS is an expensive endeavor. Direct air capture, since you’re targeting diluted CO2 in the atmosphere, is even more expensive. Securing a stable source of revenue can help de-risk investment and strengthen project viability.
Q: Are there other benefits of combining DAC with EOR?
A: The produced oil has a much lower carbon footprint than conventional sources, so long as DAC, or in some instances industrially sourced CO2, replaces the ‘naturally-sourced” CO2 discussed earlier. This could be beneficial as we find ourselves managing emissions associated with committed fossil usage over the next 2 decades. The key thing about CO2-EOR is that it uses a lot of the same infrastructure that we could easily use for geological storage. Pipelines work for both goals. Injection wells can work for both goals if designed properly. If you look at Texas and you look at where these EOR operations exist, there are plenty of saline storage sites, sometimes right below EOR fields. So there could be an opportunity to repurpose a tremendous amount of infrastructure, allowing funding to address other critical areas of climate mitigation.
Q: Shouldn’t we be aiming for underground storage instead of EOR?
A: Yes, entirely. But the argument is not as simple as choosing between Door A (storage) and Door B (EOR). There is a bit of nuance involved, unfortunately.
In reality, as evidenced by the current status of CCS, CO2-EOR plays heavily into project viability. Hence, you can’t simply assume that if you were to choose storage that the project would have proceeded as planned. Perhaps storage was never an option because of economics or risk or readiness. So the question becomes: can we wait for storage? Or do we want something more immediate and use CO2-EOR as a financial mechanism to enable deployment of these technologies and send them down their learning curves? It is important that we understand the climate implications of both pathways.
Q: Why can’t we just bury the CO2 now?
A: Two reasons, primarily. First, what are the economics for storage? In CO2-EOR, you’re producing a fuel. You have a revenue stream because CO2 is an operational expense. What is the incentive for storage today? That mainly comes from 45 Q which just benefitted from a huge boost in the Inflation Reduction Act. 45 Q will pay $180 per ton for that DAC CO2 sent to storage, or $135 for DAC/EOR or other beneficial reuse. But there is also revenue to consider. EOR will pay for that CO2 as an operational expense.
Previously, the gap was $50 for storage, $35 for EOR. The new amendments have the potential to flip those economics, and we hope this leads to the increased viability of projects tied to storage.
But there are also geophysical limitations. We need to make sure that those sites are safe and suitable for storage. For example, the Safe Drinking Water Act stipulates that if you’re injecting CO2 underground, you must monitor and characterize that site. You’ll also need something known as a Class VI well, and these are particularly hard to come by. Permitting and approvals can lead to long lead times for storage projects. So one of the best things we can do is invest in faster Class VI permitting.
Q Back to the big picture, how much direct air capture will we need?
A: The amount of direct air capture we need is certainly contested. Some say we need none: that we can achieve our goals entirely through nature-based CDR solutions like afforestation, reforestation, improved forest management, soil sequestration, regenerative agriculture, etc. There are a lot of benefits to these types of solutions, particularly to ecosystems and biodiversity. But in terms of permanence and durability of that stored CO2, these projects often fail to add up and have lately come under increased scrutiny.
We have to keep our eye on the prize here. We are trying to lower and limit atmospheric CO2 accumulation and concentration. Engineered solutions really offer the security of long-term storage that is much more challenging to achieve in the nature-based alternatives. But we shouldn’t fall into the trap of thinking any one solution will get us there. We will need a portfolio of nature-based solutions and engineered solutions like DAC. So, say conservatively we’ll need DAC to take on 10 percent of that portfolio. That would still place us at the billion-ton per year mark.
Where are we today? The largest DAC plant removes 4,000 tonnes of CO2 from the air per year. So, we’re looking at an enormous growth, decade on decade to reach that gigaton level by mid-century. That road will be paved with some very challenging problems, decisions and tradeoffs. That’s why it is so necessary to study how DAC/EOR pathways play into that future.
Interested in hearing more? Listen to the episode of the Energy Policy Now that explores this topic.
Andy Stone
Energy Policy Now Host and ProducerAndy Stone is producer and host of Energy Policy Now, the Kleinman Center’s podcast series. He previously worked in business planning with PJM Interconnection and was a senior energy reporter at Forbes Magazine.
Peter Psarras
Research Assistant Professor of Chemical EngineeringPeter Psarras is a research assistant professor in chemical and biomolecular engineering at the School of Engineering and Applied Sciences and the Kleinman Center for Energy Policy.