
Storing CO2 in Industrial Waste Materials for Scalable Climate Mitigation
Industrial waste could be a scalable solution for carbon storage, with billions of tons of COâ‚‚ storage potential locked within byproducts like mining waste and steel slag.
Industrial waste—often viewed as a costly environmental burden—could play a critical role in tackling climate change. Materials like coal ash, steel slag, and mine tailings hold immense potential for carbon storage through mineral carbonation. The origin and type of industrial waste depend on the parent process: mine tailings are rich in rejected mineral phases from metal extraction, cement kiln dust results from lime making, and steel slag is created during stainless steel production. The United States houses 2 billion tonnes of coal ash stored in 1,000 impoundments and billions of tonnes of legacy mining waste.
In addition to providing critical minerals, many of these legacy waste materials can also be used to durably store CO2. The carbon storage potential of industrial byproducts is estimated between 2.9 and 8.5 billion tonnes per year by 2100, depending on the increase in industrial waste production to supply the energy transition.
Mineral carbonation is a natural process where minerals react with CO2 to form carbonate minerals. While this occurs over millennia, the urgency of our current climate crisis demands a much faster solution. Scientists have developed methods to accelerate this process, reducing the timescale from millennia to weeks or hours.
Mineral carbonation can be achieved through three primary pathways: enhanced rock weathering, in-situ mineralization, and ex-situ mineralization.
Enhanced Rock Weathering (ERW): ERW involves crushing reactive minerals and spreading them over farmlands, where they dissolve and draw CO2 into the local watershed. Penn’s Clean Energy Conversions Lab previously conducted outdoor experiments to evaluate the potential carbon uptake of such systems. A significant focus of ERW research is understanding the duration and certainty of carbon storage in waterways and oceans, ensuring long-term effectiveness.
In-Situ Mineralization: This method involves injecting CO2 into basaltic rock formations located more than 800 meters below the Earth’s surface. At these depths, CO2 exists in a supercritical phase, occupying only 4% of the volume it would at surface conditions. This phase not only allows for efficient storage but also enhances the density and stability of the stored carbon.
Ex-Situ Mineralization: This approach involves using chemical reactors to react CO2 with minerals outside their natural environment. This method enables reactors to be strategically placed near legacy material stockpiles, like mines and coal plants. The resulting carbonate minerals can be used as feedstocks in critical supply chains, such as construction materials, promoting a circular economy and improving process economics. A significant co-benefit of this approach is the ability to separate other valuable critical minerals and rare earth elements from the feedstock within the processing of alkalinity extraction.
All mineral carbonation technologies aim to store CO2 in minerals. Rocks and CO2 are always present, but system configurations depend on local resources and constraints. For ex-situ mineralization, CO2 needs to be captured and rocks need to be mined: industrial byproducts can replace minerals to minimize mining. A geographic analysis of CO2 sources and industrial waste materials in the U.S. shows that these waste products may provide durable carbon storage in regions where subsurface storage is not possible.
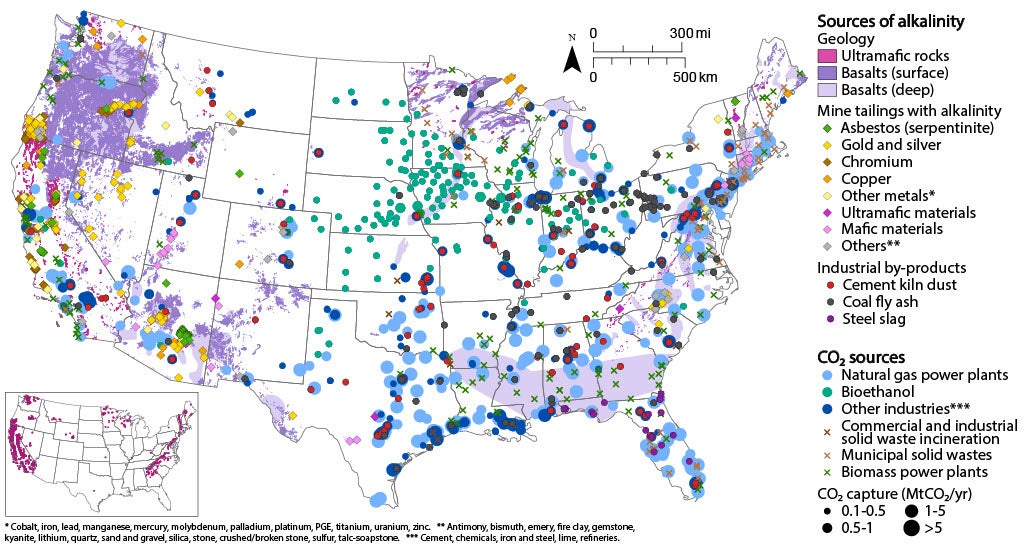
Katherine Vaz Gomes
Student Advisory Council MemberKatherine Vaz Gomes recently defended her Ph.D. in chemical engineering at the University of Pennsylvania. She is a graduate research assistant in the Clean Energy Conversions Lab and a member of the Kleinman Center’s Student Advisory Council.
Hélène Pilorgé
Research Associate, Clean Energy Conversions LabHélène Pilorgé is a research associate with the University of Pennsylvania’s Clean Energy Conversions Laboratory. Her research focuses on carbon accounting of various carbon management solutions and on Geographic Information Systems (GIS) mapping for responsible deployment of carbon management.
Likhwa Ndlovu
Ph.D. Student, Chemical and Biomolecular EngineeringLikhwa Ndlovu is a first-year Ph.D. student in the Department of Chemical and Biomolecular Engineering at the University of Pennsylvania. His research focuses on leveraging geoscience principles for the responsible deployment of carbon management solutions.
Olivia Schumacher
Undergraduate Student, University of MichiganOlivia is an undergraduate student at the University of Michigan, studying Neuroscience with the Class of 2026. She joined the Clean Energy Conversions Lab at Penn as an intern, specializing in the intersection of environmental justice and carbon sequestration.
Jennifer Wilcox
Presidential Distinguished ProfessorJen Wilcox is Presidential Distinguished Professor of Chemical Engineering and Energy Policy. Her research expertise is in carbon capture and sequestration technologies, in order to minimize the negative impacts of fossil fuels.