Remote Sensing-Based Monitoring Networks for the Next Generation of Energy and Environmental Policymaking
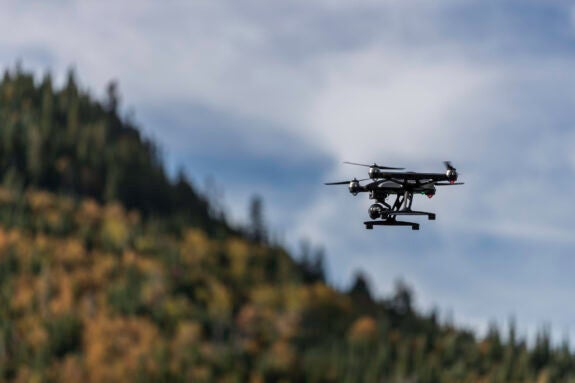
Revolutions in remote sensing technologies and data science offer the potential to transform environmental policymaking and meet the existential challenges of climate change, biodiversity loss, and pollution.
At a Glance
Key Challenge
The world needs to develop policies that address the complex nature of environmental degradation and contribute to the transformational change needed to achieve sustainability.
Policy Insight
Properly designed environmental monitoring systems can improve not only policymaking but also transparency, public understanding and empowerment, environmental justice, and government accountability.
Executive Summary
The current revolutions in remote sensing technologies, electronics, computing, and data sciences are rapidly transforming our ability to gather, analyze, and integrate environmental data for environmental policymaking.
New observational platforms and monitoring systems provide a rapidly growing array of environmental monitoring data with improving spatial and temporal scales, granularity, and sensitivity. New machine learning and artificial intelligence techniques allow for the ingestion and rapid analysis of that data. New networking capabilities enable data to be integrated and made accessible to users, stakeholders, and the public.
These advancements and the insights they enable, if they can be leveraged effectively, present the opportunity to significantly improve the ability of environmental policymaking to meet the existential challenges of climate and environmental degradation, while fostering citizen engagement.
Doing so will require policymakers to embrace new, evidence-based approaches to policy development; develop more remote sensing platforms that expand capabilities to collect meaningful data; build the capacity of public agencies to analyze and use data; and provide various publics not only with access to data but also the capacity to do independent analyses of it to support their own efforts.
The strengths and limits of current technology and current examples of such monitoring initiatives are discussed to illustrate what is possible if these powerful new technologies and systems can be networked and fully leveraged by policymakers.
In its 2019 assessment of global conditions, the United Nations Global Environment Programme (UNEP) found that the world faces triple planetary crises of climate change, biodiversity loss, and pollution. Further, UNEP said that the situation is deteriorating and the window for action is closing, finding that policies cannot keep pace with the current rate of environmental degradation (UN Environment, 2019).
UNEP called for the development of “policies which address the systems that are the root cause of environmental degradation” and that “contribute to the transformational change needed to achieve an environmentally sustainable world by 2050.”
The World Economic Forum’s 2024 Global Risks Report finds that trajectory unchanged, identifying the top four risks facing society over the next decade as extreme weather, earth systems change, biodiversity collapse, and natural resource shortages (World Economic Forum, 2024).
Clearly, the world has yet to develop and implement smart, robust energy and environmental policies that effectively address these triple planetary crises. To be sure, a lack of political will and the power of incumbent industries and interests are at the root of this existentially dangerous shortfall, and are the first hurdles that must be overcome. There is no substitute for doing so. But what other policymaking changes are needed?
The current revolutions in electronics, computing, remote sensing technologies and data sciences present the opportunity to significantly improve the ability of environmental policy to meet the existential challenges of climate and environmental degradation, if they can be leveraged effectively.
Satellites have changed the way we observe the earth (Zhao et al., 2022). New, higher quality sensor devices provide the ability to monitor and measure environmental conditions at high-resolution and in real-time, and over large scales. New machine learning and artificial intelligence techniques create the ability to ingest huge volumes of data of different varieties and from different sources and allow more rapid and accurate analysis and derivation of insights. New networking capabilities enable data from a variety of sources to be integrated and made accessible to governments, the scientific community, stakeholders, and the public.
These advances, taken together, create an opportunity to build more sophisticated evidence-based decision-making systems that can support the design of improved environmental policy, monitor compliance with those policies, and conduct more effective enforcement activities. (See Technology Overview.)
Remote sensing tools allow data gathering over large scales and at increasing resolution as technology advances. Artificial intelligence (AI) and machine learning (ML) techniques can process the large volumes of data those tools generate to extract insights with improving accuracy and reliability. Examples of the kind of rapidly growing advances enabled by this potent combination of technologies are introduced in this paper.
It is equally important for policymakers to understand the current limitations of remote sensing technologies and their associated data tools for their effective application in policy design and implementation, and to set an agenda for their continued improvement. Those current limitations, too, are introduced briefly below.
Remote Sensing
Remote sensing devices collect data about an object, place, area, or phenomenon at a distance (Testbook 2023). They have become indispensable tools in monitoring various aspects of environmental systems. These devices utilize a range of technologies and platforms, including satellite photography and hyperspectral imaging, aerial photography, lidar, radar, and airborne (plane, helicopter, drone, or balloon) sensors. They are used to collect data on factors such as weather, air pollution, land use, vegetation health, carbon storage, air quality, and soil condition.
Remote sensing devices have limitations with detection range (Perrone et al. 2023) and temporal frequency (Li et al. 2014), and are impacted by atmospheric conditions (Thompson et al. 2018). Satellites and airborne sensors capture information on specific characteristics over broad areas; other devices like drone- or aircraft-mounted sensors may have higher resolution but are much more limited in the aerial extent of coverage.
Remote sensing observations involve massive amounts of data that must be processed and stored (Ma et al. 2015) and require highly developed algorithms that must still be ground-truthed (Loew et al. 2017).
The costs of these technologies vary widely. Satellites, especially, are costly to develop and deploy, and have long lead times. This can present financial barriers to their accessibility, which has equity implications (Turner et al. 2015).
The choices remote sensing users make when using and interpreting data can have material and potentially lasting impacts on people and ecologies, and raise ethical and justice questions (York et al. 2023), as well as questions of impacts on individual privacy, civil liberties, and national security (McKenna et al. 2019).
Artificial Intelligence and Machine Learning
The integration of Artificial Intelligence (AI) and Machine Learning (ML) in environmental monitoring has seen rapid advancements (Hickmon et al. 2022), enabling the processing of large volumes of satellite data efficiently and the extraction of valuable insights from large datasets.
AI and ML algorithms are employed to identify patterns, anomalies, and relevant features in the data that might be challenging for traditional methods (Belgiu and Drǎguţ 2016). ML techniques are utilized for satellite image classification, for example, to automatically categorize different land cover types, aiding in monitoring changes in vegetation, urban areas, and other environmental features (Loussaief and Abdelkrim 2016).
AI is used, for example, with satellite observations in climate modeling; ML algorithms can analyze historic climate data to predict future trends (Schneider et al. 2023).
AI is used with satellite imagery to monitor environmental disasters like wildfires, floods, and earthquakes. ML algorithms are used to analyze the extent of damage and aid in disaster response planning (Ghaffarian et al. 2023).
Satellite data, combined with AI, is used for ocean and atmospheric research. ML algorithms are used to analyze complex interactions within these systems to improve weather forecasting and climate modeling (Loeb et al. 2021).
AI and ML, too, have limitations that impact their ability to provide accurate and reliable information. Environmental systems are complex and highly variable, and can change suddenly, limiting AI’s ability to accurately represent the interconnected nature of systems (Cortés et al. 2000), their predictive use (Willard et al. 2023), and reliability in modelling (Irrgang et al. 2021). Data gaps or inaccuracies can also impact model reliability (Jordan and Mitchell 2015). The extent to which AI models are able to holistically integrate various scientific disciplines can limit their robustness (Huntingford et al. 2019) and adaptability (Maganathan et al. 2020). The energy demands of AI and ML applications have climate impacts (Kaack et al. 2022).
In environmental monitoring, transparency is crucial for informed decision-making, and the often “black box” nature of AI models and lack of interpretability and explainability is a significant limitation (Haresamudram 2023). AI models also may not fully capture the social impact of certain policies or the ethical considerations associated with specific environmental interventions. Additional concerns related to privacy, data ownership, and potential misuse of technology need careful consideration (Nassar and Kamal 2021).
Understanding and addressing these limitations is crucial for ensuring the responsible and effective use of AI and ML in monitoring applications and policy application.
Application Examples
- NISAR. NASA’s joint mission with the Indian Space Research Organisation (ISRO) will launch in 2025 to measure Earth’s changing ecosystems, dynamic surfaces, and ice masses on a 12-day cycle, providing information about biomass, natural hazards, sea level rise, and groundwater; and supporting other applications (NASA n.d.).
- PACE. NASA’s Plankton, Aerosol, Climate, ocean Ecosystem satellite, launched in February, 2024 and will study ocean health, air quality, and the effects of climate change (Dooren 2024).
- Global Monitoring Laboratory (GML). This National Oceanic and Atmospheric Administration (NOAA) effort uses satellite and other data sources to conduct research on greenhouse gas and carbon cycle feedbacks, changes in clouds, aerosols, and surface radiation, and recovery of stratospheric ozone (NOAA n.d.).
- Planet. This private company combines ecosystem science, high-frequency, high-resolution satellite-based earth observation (EO), and AI, derive insights (Planet n.d.), including a global-scale, high resolution AI-powered forest carbon monitoring system (Business Wire 2024) and a project to monitor global biodiversity hotspots (Planet 2024), both of which were launched in 2024.
- Skytruth. This NGO uses satellite data and analysis for environmental protection initiatives (SkyTruth 2024).
- Global Forest Watch. Established by NGO World Resources Institute, GFW uses satellite data and other tools to generate data for monitoring forests (Global Forest Watchd.).
- Earth Genome. This NGO combines satellite data, artificial intelligence, and machine learning to “guide and measure effective actions on the most pressing climate and conservation issues” (Earth Genome d.). Its products include:
- CTrees uses cloud-based computing and AI to integrate advanced satellite technology and other data to accurately map variations of carbon stored in forest and non-forest landscapes. (Earth Genome d.)
- Earth Index uses satellite imagery, multispectral data, computer vision models, and machine learning to identify landscape-level changes (Earth Genome d.).
- Synoptic. This public benefit corporation, aggregates, processes, and distributes real-time and historical weather and environmental observation data (Synoptic n.d.).
Examples of evolving integrated monitoring platforms include:
- Global Environment Monitoring. UNEP’s platform provides integrated data on global environmental conditions (UNEP n.d.).
- Integrated Carbon Observation System (ICOS). This European Union initiative produces “standardized, high-precision and long-term observations” on the carbon cycle and greenhouse gases (ICOS n.d.).
Evidence-based Policymaking
A 2017 report from the U.S. Federal Commission on Evidence-Based Policymaking presaged UNEP’s call for more effective policymaking:
Policymakers must have good information on which to base their decisions about improving the viability and effectiveness of government programs and policies. Today, too little evidence is produced to meet this need.
The Commission…envisions a future in which rigorous evidence is created efficiently, as a routine part of government operations, and used to construct effective public policy. The Federal government has already taken important steps towards accomplishing this vision, but much work remains.
Modern technology and statistical methods, combined with transparency and a strong legal framework, create the opportunity to use data for evidence building in ways that were not possible in the past. (Commission on Evidence-Based Policymaking 2017)
Two key issues in environmental policymaking illustrate the opportunities to leverage the technology and scientific revolutions to obtain better information and analysis, and to design more effective policies.
First is the scale of policymaking. While environmental data gathering has advanced markedly in recent decades, persistent environmental data gaps still impair the ability to design policy at more effective scales, adversely affecting the public and the environment. (See Air Pollution.)
The Federal government sets concentration standards for principal air pollutants—carbon monoxide, lead, nitrogen dioxide, ozone, particulate matter, and sulfur dioxide—to protect public health and to prevent environmental damage (Pennsylvania DEP n.d.). State and sub-state entities1 monitor these principal pollutants using a combination of continuous automated monitoring stations and manual sampling.
The limited number of stations generally allows for regional-level air quality assessments. For example, PM2.5 monitors are required by the U.S. Environmental Protection Agency (EPA) in each metropolitan statistical area (MSA) over 500,000 in population, as well as many lower population areas. For MSAs with a population of a million or more people, one additional monitor must be located at a near-road site, and for some locations, an additional monitor is required in an area of poor air quality (U.S. EPA 2024).
Emissions estimates are calculated from this data and feed into the National Emissions Inventory (NEI) (U.S. EPA n.d.). That data feeds EPA’s AirNow (U.S. EPA n.d.), which provides air quality data at local, state, national, and world levels on an hourly basis.
To be sure, AirNow is an indispensable and continually improving tool. However, accurate, real-time data on some sources of pollution, such as small industrial facilities, are lacking.2 The fact that exposure to air contaminants is sub-local—indeed, it is personal—has major implications for the ability of public policy made on a regional scale to address health impacts and environmental justice issues.
The granularity of current estimates of air pollution could be enhanced with a combination of more localized monitoring and higher-resolution remote sensing-based monitoring. Technology is moving rapidly in the direction of this capability, and presents an opportunity to enable more protective policies and other interventions to be developed at sub-regional, i.e. more local, scales and improve environmental outcomes.
Recent Developments:
- Tropospheric Emissions Monitoring of Pollution (TEMPO). In April, 2024, NASA launched TEMPO, an instrument that monitors major air pollutants (primarily nitrogen dioxide, formaldehyde, and ozone) on an hourly basis at high spatial resolution in North America (Atkinson 2023). TEMPO will provide data on how pollution varies by neighborhood within a city, and will be especially useful in examining issues of environmental justice. This tool will join satellites from South Korea and the European Space Agency in a global constellation of satellites gathering data on air quality (TEMPO 2023).
- Greenhouse Gas and Air Pollutants Emissions System (GRA2PES). The Chemical Science Laboratory of the National Oceanic and Atmospheric Administration (NOAA) and National Institute of Standards and Technology (NIST) Greenhouse Gas Measurements Program of the U.S. Department of Commerce are working to develop a new capability to measure and model national emissions of greenhouse gases and hazardous air pollutants to support air quality and greenhouse gas mitigation sectors (NIST n.d.).
- IQAir. This commercial application collects data from a network of ground-based sensors worldwide, and applies artificial intelligence and machine learning to provide air quality information (IQAir n.d.).
A second issue facing current energy and environmental policymaking is overreliance on industry self-generated data. The case of methane emissions from the oil and gas sector is a particularly important example. (See Methane Emissions from the Oil and Gas Sector.)
Methane is a powerful, heat-trapping greenhouse gas that is the second biggest contributor to human-caused global warming after carbon dioxide (IEA 2024). Over a 20-year period, methane is about 80 times more potent than CO2 at forcing climate warming (UNEP 2021). According to the International Energy Agency (IEA), methane is responsible for about 30% of the rise in global temperatures since the industrial revolution (IEA 2022), and methane emissions from oil and gas operations must be “the first to go in limiting warming in the near-term” (IEA 2024).
Reducing fugitive methane emissions, to a significant degree, requires the industry to adopt well-known solutions, such as leak detection and repair regimes, electrification of equipment, and other practices that are still more the exception than the norm. Crafting the right policy interventions and regulations to ensure that adoption, with the appropriate levels of stringency and urgency, requires a well-developed understanding of the size and scale of the problem to be solved.
Current policies lack that understanding because we really don’t know exactly how much methane the oil and gas industry actually emits. Official government estimates of methane emissions are based on summations of industry self-reported engineering calculations (Heath et al. 2015)—not real-time monitoring.
Numerous studies and meta-analyses (Zhang et al. 2023) have attempted to estimate actual oil and gas methane emissions, using a wide variety of detection equipment and methodologies. They have generally found that methane emissions from the oil and gas sector are far greater than what companies—and hence governments—report. One recent study found that methane emissions from the United States oil and gas fields were roughly three times the national government inventory estimate (Sherwin et al. 2024). Another study using data collected from aircraft-mounted sensors that were flown over regions accounting for 70% of onshore U.S. oil and gas production showed that methane emissions are over four times higher than EPA estimates (MethaneSat 2024). Emissions from offshore oil and gas production and storage are even less well understood (Krishnan 2024).
Recent Developments:
Technology
There is a growing ecosystem of satellite observations of methane emissions, including:
- Copernicus Sentinel-5P. This European Space Agency satellite maps global methane concentrations on a daily basis and enables the detection of large methane sources (ESA 2023).
- GHGSat.This privately-owned Canadian company uses high-resolution satellites and proprietary emission sensing technology to monitor methane and CO2 emissions (GHGSAT n.d.).
- Climate TRACE. This non-profit coalition uses satellites, other remote sensing techniques, and artificial intelligence to create a facility-level global inventory of individual sources of emissions and mapping the 70,000 highest emitting greenhouse gas sources (Climate TREACE n.d.).
- Carbon Mapper. This NGO uses satellite and airborne monitoring technology to identify methane and CO2 emissions at a facility-scale (Carbon Mapper n.d.), and in August, 2024, in collaboration with satellite data company Planet, launched its first hyperspectral satellite to detect and track methane and CO2 super-emitters at a level of granularity that can support direct mitigation action (Business Wire 2024).
- Mineral Dust Source Investigation (EMIT). This National Aeronautics and Space Administration (NASA) mission has the ability to identify “high-confidence research grade methane plume complexes from point source emitters” and provides that data through its Open Data Portal (NASA n.d.).
- Methane Alert and Response System (MARS). UNEP uses satellite data to detect very large methane emissions around the world (UNEP n.d.).
- MethaneSAT. This Environmental Defense Fund satellite launched in 2024 and will be fully operational in 2025. It will collect data on atmospheric concentrations of methane to identify high emitting point sources (Liao 2024).
- U.S. Greenhouse Gas Center. This is a multi-agency Federal effort whose goal is “to provide decision makers with one location for data and analysis” (U.S. Greenhouse Gas Center n.d.). It provides open access to data on greenhouse gases that consolidates greenhouse gas information from observations and models to create a unified source for U.S. government and other datasets.
Policy:
- The Inflation Reduction Act of 2022 (IRA). This law required EPA to revise emissions calculation methodologies for existing and newly-identified sources of emissions, driven in part by data from atmospheric observations. Those updates were finalized in May, 2024 (U.S. EPA 2024).
- Waste Emissions Charge (WEC). The IRA also required EPA to impose an annual Waste Emissions Charge for methane emissions that exceed specified waste emissions thresholds from certain oil and gas facilities. The charge is based on emissions calculations and was finalized in November, 2024. (U.S. EPA 2024).
- Regulations Embrace Methane Detection Technologies and Tools. On March 8, 2024, EPA published its final rule targeting methane emissions from the oil and gas sector (Federal Register 2024). The rule embraces recent advances in new methane detection technologies and tools to model to the effectiveness of those technologies in leak detection and repair (LDAR) programs at oil and gas facilities. Those same tools aid oil and gas companies in optimizing their investments in deploying these detection technologies (Ravikumar n.d.).
- Super Emitter program. Among other provisions, the new 2024 methane rule creates the “Super Emitter” program designed to identify and address significant methane leaks from production facilities, including an avenue for qualified third parties using remote sensing technologies (satellites or aerial surveys only) to alert EPA of entities exceeding the emissions standards and for the EPA to require owners/operators to investigate such alerts. The rule also allows regulated entities the option to replace traditional LDAR programs with advanced measurement technologies such as on-site sensor networks or aerial flyovers using remote sensing.
- Incorporating satellite data in reporting. In August 2024, EPA solicited additional information on how to incorporate data from satellites and other advanced measurement technologies into its U.S. Greenhouse Gas Reporting Program (EPA 2024).
- European Union (EU) methane rules. In August 2024, the European Union’s first-ever rules to curb methane emissions from the energy sector became effective. The new regulation requires the European oil and gas industry, as well as the coal industry, to measure, monitor, report and verify their methane emissions and to take action to reduce them. The regulation will progressively require exporters to the EU to apply the same monitoring, reporting and verification obligations as EU operators (EU 2024).
The regulation also contains measures aimed at increasing transparency on methane emissions from imported oil, gas and coal (IEA 2024), including:
- Establishment of a public methane transparency database of emissions reported by importers and EU operators
- Creation of country and company methane performance profiles for importer use
- Implementation of a global methane emitters monitoring system, based on satellite data, and rapid alert mechanism for super-emitting events within or outside the EU.
- Requirement that new import contracts for oil, gas, and coal mandate equivalent MRV requirements for exporters as those established for EU producers, beginning in January 2027.
Because Europe imports the majority of the fossil fuels it consumes (Wettengel 2024), these regulations will also help to reduce methane emissions from imported fossil fuels produced around the globe. The United States is a key supplier of oil and natural gas to the EU. In the first quarter of 2024, 17.1% of the EU’s oil imports came from the U.S. (Eurostat 2024). In 2023, the US was the largest supplier of LNG to the EU, providing nearly half of all imports (U.S. EIA 2024).
- U.S.-led shared global framework for estimating GHG emissions. The U.S. Department of Energy is working with major LNG importing and exporting countries to develop a shared global framework for estimating GHG emissions across the natural gas supply chain (DOE 2024). Current life cycle analysis models of US LNG supply chains are based on emissions estimates in national inventories that, as discussed here, have been demonstrated to significantly underestimate emissions (Zhu et al. 2024).
- Coalition for LNG Emission Abatement Toward Net Zero. Utilities and trading houses in Japan and South Korea are joining a Coalition for LNG Emission Abatement Toward Net Zero initiative that aims to leverage their buying power to curb methane emissions from LNG supply chains (Oda and Inajima 2024). Japan and South Korea are the second- and third-largest buyers of LNG and together accounted for 27% of the world’s imports of the fuel last year (IEEFA 2024).
The estimation improvements, use of remote sensing data, and expanded use of satellite data are critically important. Reliance on engineering calculations to assess methane emissions, industry performance, and compliance should be replaced as soon as technology allows3 with the establishment of methane emissions monitoring based on combinations of fixed (continuous monitoring systems) and mobile (vehicle-, drone-, plane- and satellite-based) methane leak detection technologies. Data gathered from such systems will provide real-time emissions data that can enable improved compliance monitoring, swifter enforcement actions, and inform the design and development of additional, targeted regulatory steps that are needed to minimize emissions from this sector.
As an added benefit, this approach to methane monitoring can also be applied to the analogous challenge of managing, minimizing, and regulating methane emissions from other industrial sources, such as landfills and coal mines. Emissions estimates for these sectors suffer from the same deficiencies, including self-reporting, as those from the oil and gas sector. Satellite measurements suggest that actual emissions from landfills could be almost double EPA estimates (EDF 2024). In Pennsylvania, for example, fugitive emissions from underground coal mines are estimated to be the second largest source of methane emissions and account for 45% of all fugitive emissions in the state (PA DEP 2023).
Technological developments in earth observation and data science now present the opportunity to gather more and better-quality data, inviting an accompanying transformation in the ways in which we measure, monitor, and understand environmental conditions, design policy, and monitor regulated community compliance (Paddock 2024).
A comprehensive, real-time earth observation and monitoring capacity, consisting of satellites, other levels of ground-based and airborne remote and in situ sensors measuring parameters like air and water quality and soil conditions, and the analytical platforms to ingest, analyze, and serve massive amounts of data, is increasingly within reach.
It is a goal that the triple planetary crises described by UNEP call us to pursue. In addition to government-deployed satellites and sensors, international, commercial, and NGO assets (some examples are described in this paper) could be leveraged to develop such systems. Data gathered by volunteer efforts like, for example, local watershed protection and environmental quality groups can be included if appropriate data quality standards can be developed and accepted. Ultimately, citizen-gathered data, data from wearable technologies (Salamone et al. 2021), and from the Internet of Things (Shah and Mishra 2016) can all be brought into such a system.
Such systems, enhanced with artificial intelligence and machine learning applications, offer the potential of turning vast amounts of data generated by these platforms into insights at scales necessary to explain complex environmental interactions and to drive solutions at local and global scales (Fernholz 2024).
A conceptual schematic of this kind of monitoring system is presented in Figure 1. There are significant issues that are beyond the scope of this paper that that would have to be managed as part of the design and development of such system. Technical issues like data standards, formats, interoperability would need to be worked out and standardized. On a systems-level scale, the sheer volume of data to be ingested, data ownership, data control, privacy, and confidentiality would need to be managed. And because earth systems are so complex and interrelated, with feedback mechanisms, various scientific disciplines and domains would need to be involved in interdisciplinary analysis and insight derivation.
It is also vitally important to consider the issues of equity and access—and the implied issue of literacy—in the design of such systems. Capacity building for multiple categories of users—all levels of governments, academia, stakeholders, and the public—should be foundational to the design and operation of these kinds of systems. The systems should be user-friendly, and the data generated from these efforts should be made freely available as broadly as possible to other levels of government, researchers, and the public as a public good.4
The cost of building, operating, and maintaining these systems is an obvious issue. Clearly, the Federal government should lead here, and partner (or continue to partner) with private companies and NGOs (some are identified in this digest) wherever possible.
Like many of the solutions that are needed to address the triple planetary crises that pass cost-benefit tests (Glanemann et al. 2020), the development of these monitoring systems is likely to be chiefly a matter of sustained political will to invest. A combination of approaches—public ownership, public-private partnerships, partnerships with academia, the development of novel business models to accommodate commercial data—will likely be needed to realize the full potential of this concept.
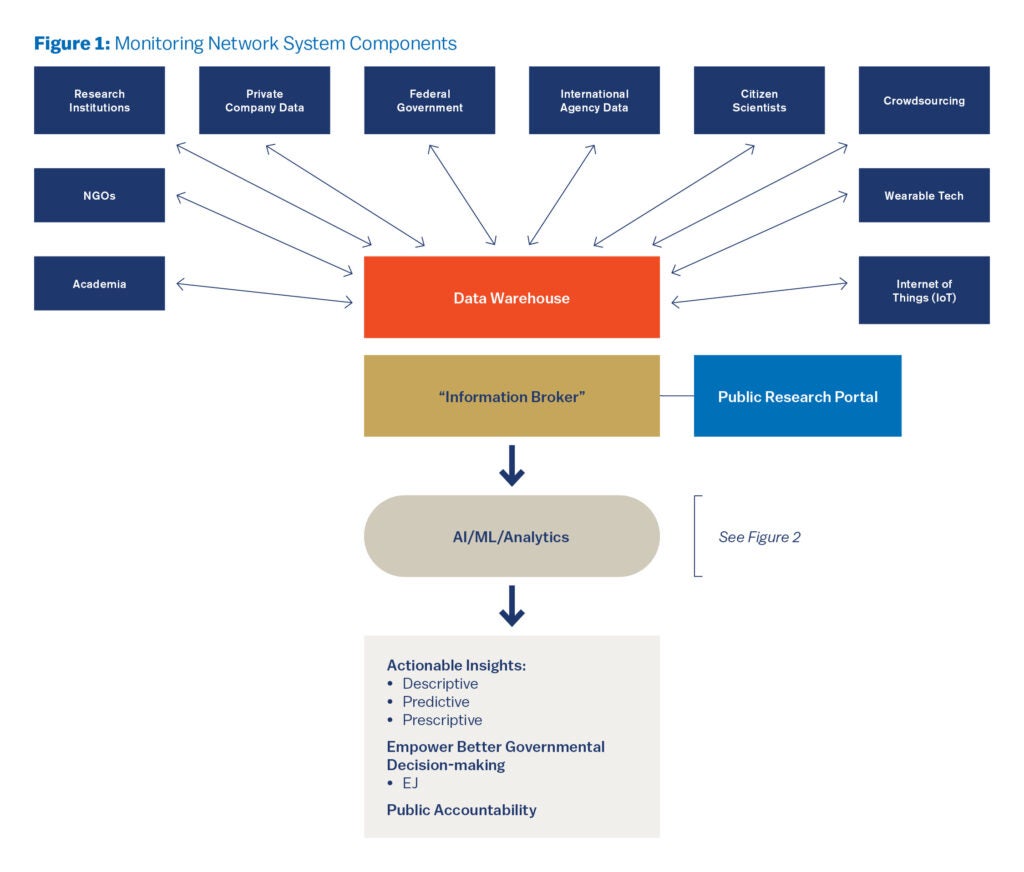
A data warehouse (Umar 2022) approach that brings together the data from multiple sources and networks and facilitates access for analysis by decision makers could be useful in the development of new analytical approaches, tools, and applications with which to derive enhanced insights. A public research portal could similarly empower the non-governmental sector and the public. See Figure 2.
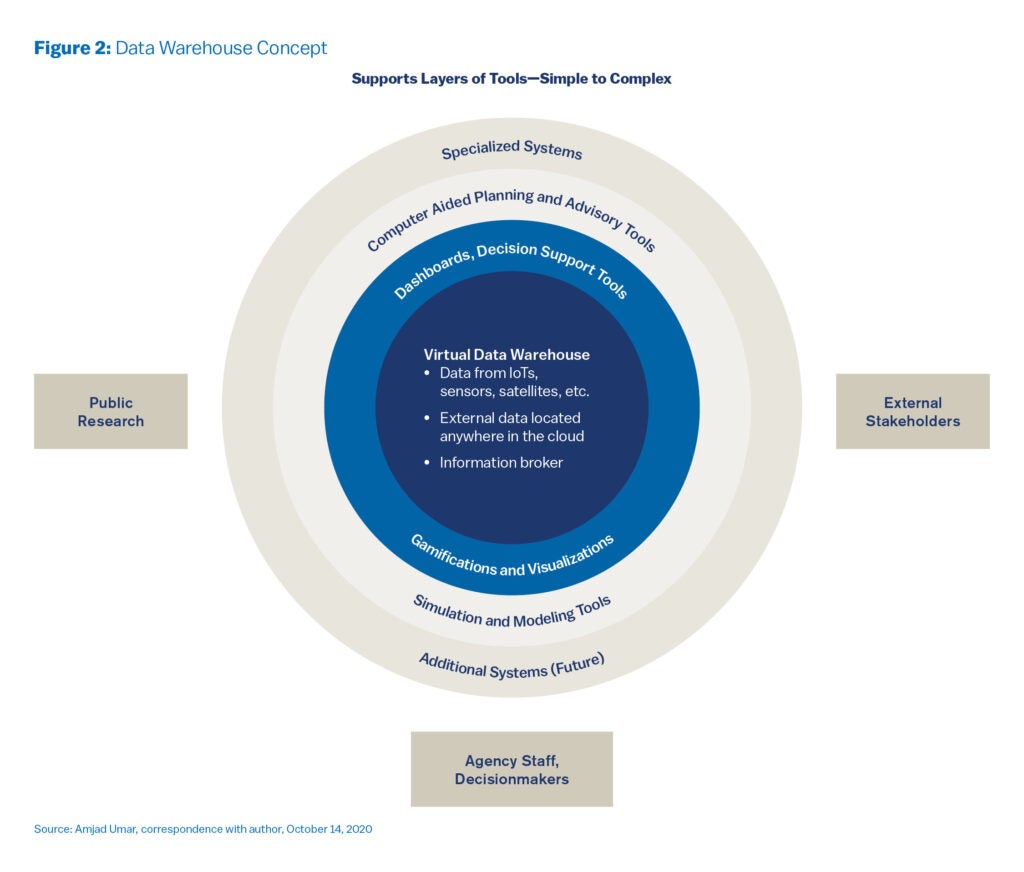
The challenges for improved policymaking are to enable and expand the use of these kinds of systems by combining and networking layers of remote sensing technologies; to propel the continued development of suites of technologies that will allow for more granular assessments; to use these systems for refined analyses of localized environmental conditions; and then to incorporate the resulting insights into policy design.
Efforts are underway across many platforms and organizations–government, NGOs, universities, and private companies—to build components of such a system. Examples are referenced throughout this report. They illustrate what is possible in discrete areas, and hint at what is possible if these powerful new systems and be networked and leveraged by policymakers.
Benefits of Next-Generation Environmental Monitoring
This next generation of environmental monitoring systems will enable:
- filling data gaps to better describe and understand complex systems and current conditions
- real-time monitoring of environmental conditions, regulated community performance, and compliance with regulatory requirements
- better prediction of changes in those conditions
- prescription of more effective and protective policies
- more targeted regulatory interventions
- more effective and comprehensive compliance monitoring and greater compliance5
- improved environmental performance and outcomes
Provided that platforms are intentionally designed to provide discoverability and easy access to the data for stakeholders and the public and include capacity-building elements such as on-line tutorials and other accessible training to enable users of all categories to understand and use the data, this approach offers other important co-benefits:
- Transparency: Making data, analyses, and methods available, accessible, and understandable to researchers, and especially to stakeholders and the public via explanation, contextualization, and capacity building, creates an opportunity for improved government transparency.
- Democratization: Data availability should be as broad as possible and could be leveraged for scientific advancement, better public understanding, and enhanced engagement in policy development. More robust data and analyses certainly will not reduce policy conflict, nor will it deter special interests from challenging the facts or fighting progress. But, properly leveraged, it could make the job of special interests more difficult (Esty and Rushing 2007).
- Understanding and Empowerment: Data made available, accessible, understandable, and usable to local governments, the public and stakeholders can build greater awareness and understanding of environmental issues and empower communities to take their own actions as well as participate more robustly in the policy process.
- Environmental Justice: More granular data and analysis will enable the development of improved cumulative impacts analysis. Cumulative impact analysis is an essential tool in environmental and public health protection, climate mitigation, and–critically–the pursuit of environmental justice (EPA 2024). This type of analysis is required of EPA by several Federal environmental statutes6, but is not always conducted at state or other levels. To be sure, remote sensing is not the answer to diagnosing all community needs and does not negate the need for other, more granular types of environmental data. Still, especially with improving measurement sensitivity of remote sensing technologies, environmental monitoring networks can empower the extraction of better insights, provide the capacity for more units of government to undertake such analyses, and improve environmental justice and related policymaking at all levels.
- Accountability: Critically, the data generated by these systems, coupled with the capacity to access, understand, and use the data, will provide the means for all stakeholders and the public to judge the effectiveness of public policy, policy makers, and regulatory entities and hold them to account.
Towards a Federal Environmental Monitoring Network
In late 2023, the Biden Administration released a report: The Federal Strategy to Advance an Integrated U.S. Greenhouse Gas Monitoring & Information System (Greenhouse Gas Monitoring & Measurement Interagency Working Group 2023), which points the Federal government squarely in the direction of the kind of monitoring network described here:
The report describes a framework for an integrated U.S. Greenhouse Gas (GHG) Monitoring & Information System (System) that takes advantage of advanced measurement and modeling capabilities, as well as the growth of GHG observational data, to provide enhanced data products. The coordinated use of atmospheric-based and activity-based approaches is expected to increase confidence in setting, assessing, and meeting climate mitigation goals…and better understand how ecosystems respond to climate warming and other human influences.
The report outlines several near-term strategies:
- Enhance and expand multi-tiered observation/measurement networks
- Accelerate the transition/scaling up of research capabilities to sustained use capability
- Enhance data products to better meet user needs
- Refine observational, modeling, and data assimilation capabilities to continuously improve future GHG measurement capabilities
- Explore options for enhancing organizational capacity to coordinate across multiple actors internal and external to government in the implementation of the above strategies
Significantly, the strategy calls for the integration of Federal and non-Federal (e.g., commercial, NGO, and citizen science) data, and transparency in both data and methods— “a systematic, unified approach to providing high-quality, transparent data and information across different geographic and temporal scales that meet user needs for actionable GHG emissions information.”
The report cites a 2022 report from the National Academies of Sciences, Engineering, and Medicine that considers machine learning and other potential advances that show promise for future system advances (NASEM 2022).
This approach to GHG monitoring should be extended and applied to other areas of environmental policymaking with an appropriate level of urgency–air pollution in general, land, water, ocean (Amos 2024) and natural resource conservation, and biodiversity, for example.
Looking Ahead
The triple planetary crises we currently face underscore the urgency of equipping policymakers with the systems and tools needed to develop evidence-based policies that can propel us to answering UNEP’s call for the transformational change that can achieve an environmentally sustainable world. Properly designed, the monitoring systems described here can improve policymaking, propel scientific advance, and offer opportunities for greater public awareness and engagement, and improve government accountability. The revolutions in remote sensing technologies and data science have made the achievement of this goal possible. This work must be pursued with the urgency that global conditions demand.
John Quigley
Senior Fellow, Kleinman CenterJohn Quigley is a senior fellow at the Kleinman Center and previously served on the Center’s Advisory Board. He served as Secretary of the PA Department of Environmental Protection and of the PA Department of Conservation and Natural Resources.
Amos, J. 2024. “How Funding Open Source Data Can Help Save the Ocean and Mitigate Climate Change.” Triple Pundit, https://www.triplepundit.com/story/2024/ocean-protection-funding/811521, accessed Oct. 7, 2024.
Atkinson, J. 2023. “New Instrument to Track Air Pollution Hourly, Shed Light on Disparities.” National Aeronautics and Space Administration, https://www.nasa.gov/missions/tempo/new-instrument-to-track-air-pollution-hourly-shed-light-on-disparities/, accessed Jan. 11, 2024.
Belgiu, M. and L. Drǎguţ. 2016. “Random Forest in Remote Sensing: A Review of Applications and Future Directions.” ISPRS Journal of Photogrammetry and Remote Sensing. https://doi.org/10.1016/j.isprsjprs.2016.01.011.
Business Wire. 2024. “Planet Releases AI-powered Forest Carbon Monitoring Product — World’s First Global Scale Forest Monitoring System at 3-Meter Resolution.” https://www.businesswire.com/news/home/20240924630576/en/Planet-Releases-AI-powered-Forest-Carbon-Monitoring-Product-.
Business Wire. 2024. “Planet to Launch Its First Hyperspectral Satellite, Tanager-1, and 36 SuperDoves with SpaceX”, August 15. https://www.businesswire.com/news/home/20240815210432/en/Planet-to-Launch-Its-First-Hyperspectral-Satellite-Tanager-1-and-36-SuperDoves-with-SpaceX.
Carbon Mapper. n.d. https://carbonmapper.org/. Accessed Jan. 9, 2024.
Climate TRACE. n.d. https://climatetrace.org/. Accessed Jan. 9, 2024.
Commission on Evidence-Based Policymaking. 2017. The Promise of Evidence-Based Policymaking: Report of the Commission on Evidence-Based Policymaking. https://bipartisanpolicy.org/download/?file=/wp-content/uploads/2019/03/Full-Report-The-Promise-of-Evidence-Based-Policymaking-Report-of-the-Comission-on-Evidence-based-Policymaking.pdf.
Cortés, U., et al., 2000. “Artificial Intelligence and Environmental Decision Support Systems.” Applied Intelligence. doi.org/10.1023/A:1008331413864.
Dooren, J. 2024. “NASA Launches New Climate Mission to Study Ocean, Atmosphere.” NASA. https://www.nasa.gov/news-release/nasa-launches-new-climate-mission-to-study-ocean-atmosphere/?linkId=314466587.
Earth Genome. n.d. “Ctrees.” https://www.earthgenome.org/work/ctrees. Accessed Jan. 10, 2024.
Earth Genome. n.d. “Introducing Earth Index.” https://www.earthgenome.org/earth-index. Accessed Jan. 10, 2024.
Earth Genome. n.d. “What We Do.” https://earthgenome.org/. Accessed Jan. 10, 2024.
Federal Register. 2024. “Use of Advanced and Emerging Technologies for Quantification of Annual Facility Methane Emissions Under the Greenhouse Gas Reporting Program.”, August 29. https://www.federalregister.gov/documents/2024/08/29/2024-19403/use-of-advanced-and-emerging-technologies-for-quantification-of-annual-facility-methane-emissions.
Environmental Defense Fund. n.d. “America’s Hidden Landfill Emissions.” https://landfills.edf.org/#americas-landfills. Accessed Oct. 8, 2024.
Esty, D. and R. Rushing. 2007. Governing By the Numbers: The Promise of Data Driven Policymaking. Center for American Progress. https://cdn.americanprogress.org/wp-content/uploads/issues/2007/04/pdf/data_driven_policy_report.pdf.
European Commission. n.d. “Methane Emissions.” https://energy.ec.europa.eu/topics/carbon-management-and-fossil-fuels/methane-emissions_en#regulation-on-methane-emissions-reduction-in-the-energy-sector. Accessed Oct. 8, 2024.
European Space Agency. 2023. “Monitoring Methane from Space.” https://www.esa.int/ESA_Multimedia/Videos/2023/11/Monitoring_methane_from_space.
Eurostat. 2024. “EU Imports of Energy Products Continue to Drop.” https://ec.europa.eu/eurostat/web/products-eurostat-news/w/ddn-20240701-1.
European Union. 2024. Regulation (EU) 2024/1787 of the European Parliament and of the Council of 13 June 2024 on the reduction of methane emissions in the energy sector and amending Regulation (EU) 2019/942. https://eur-lex.europa.eu/legal-content/EN/TXT/PDF/?uri=OJ:L_202401787.
Federal Register. 2024. Standards of Performance for New, Reconstructed, and Modified Sources and Emissions Guidelines for Existing Sources: Oil and Natural Gas Sector Climate Review. https://www.govinfo.gov/content/pkg/FR-2024-03-08/pdf/2024-00366.pdf.
Fernholz, T. 2024. “Understanding AI’s Impact on Space Data with Planet’s Head of Product.” https://payloadspace.com/understanding-ais-impact-on-space-data-with-planets-head-of-product/. Accessed Feb. 5, 2024.
Ghaffarian, S. et al. 2023. “Explainable Artificial Intelligence in Disaster Risk Management: Achievements and Prospective Futures. International Journal of Disaster Risk Reduction, Volume 98. https://doi.org/10.1016/j.ijdrr.2023.104123
GHGSAT. n.d. Greenhouse Gas Emissions Monitoring, https://www.ghgsat.com/en/. Accessed Jan. 9, 2024.
Glanemann, N. et al. 2020. “Paris Climate Agreement Passes the Cost-Benefit Test. Nature Communications. https://doi.org/10.1038/s41467-019-13961-1.
Global Forest Watch. n.d. https://www.globalforestwatch.org/. Accessed Oct. 1, 2024.
Greenhouse Gas Monitoring & Measurement Interagency Working Group. 2023. The Federal Strategy to Advance an Integrated U.S. Greenhouse Gas Monitoring & Information System. https://nspires.nasaprs.com/external/viewrepositorydocument/cmdocumentid=923603/solicitationId=%7BDDD1BC857BDDD1BC85-9276-8FB7-C362-A00E3E427E0D%7D/viewSolicitationDocument=1/GHGMIS%20Draft%20Strategy%20for%20Public%20Comment.pdf.
Haresamudram, K. et al. 2023. “Three Levels of AI Transparency.” Computer. doi: 10.1109/MC.2022.3213181.
Heath, G. et al. 2015. Estimating U.S. Methane Emissions from the Natural Gas Supply Chain: Approaches, Uncertainties, Current Estimates, and Future Studies. NREL. https://www.nrel.gov/docs/fy16osti/62820.pdf.
Hickmon, N. et. al. 2022. Artificial Intelligence for Earth System Predictability. U.S. Department of Energy. https://publications.anl.gov/anlpubs/2022/09/177828.pdf.
Huntingford, C., et al. 2019. “Machine Learning and Artificial Intelligence to Aid Climate Change Research and Preparedness.” Environmental Research Letters. DOI 10.1088/1748-9326/ab4e55.
Institute for Energy Economics and Financial Analysis. 2024. “Global LNG Outlook 2024-2028.” https://www.energy.gov/sites/default/files/2024-06/067.%20IEEFA%2C%20Global%20LNG%20Outlook%202024-2028.pdf.
Integrated Carbon Observation System. n.d. “Our Mission.” https://www.icos-cp.eu/about/icos-in-nutshell/mission. Accessed Oct. 1, 2024.
International Energy Agency. 2024. “EU Methane Regulations.” Last updated June 19, 2024. https://www.iea.org/policies/18209-eu-methane-regulations.
International Energy Agency. 2024. “Methane Abatement.” Last update on April 25, 2024. https://www.iea.org/energy-system/fossil-fuels/methane-abatement.
International Energy Agency. 2022. “Methane and Climate Change.” https://www.iea.org/reports/global-methane-tracker-2022/methane-and-climate-change.
International Energy Agency. 2024. “Methane Emissions.” Last update on April 25, 2024. https://www.iea.org/energy-system/fossil-fuels/methane-abatement.
IQAir. n.d. “AirVisual Platform.” https://www.iqair.com/us/commercial-air-quality-monitors/api. Accessed Sept. 30, 2024.
Irrgang, C, et al. 2021. “Towards Neural Earth System Modelling by Integrating Artificial Intelligence in Earth System Science.” Nature Machine Intelligence. https://doi.org/10.1038/s42256-021-00374-3.
Jordan, M. I. and T. M. Mitchell. 2015. “Machine Learning: Trends, Perspectives, and Prospects.” Science. https://doi.org 10.1126/science.aaa8415.
Kaack, L.H. et al. 2022. “Aligning Artificial Intelligence with Climate Change Mitigation.” Nature Climate Change. https://doi.org/10.1038/s41558-022-01377-7.
Klemmer, K. and E. Rolf. 2024. “Satellite Images Reveal Untracked Human Activity on the Oceans.” Nature. https://doi.org/10.1038/d41586-023-03983-7.
Krishnan, A. 2024. “Measuring Marine Methane: Understanding the Challenges of Monitoring Methane Emissions from Offshore Gas Production and Transportation.” SkyTruth. https://skytruth.org/2024/07/measuring-marine-methane-understanding-the-challenges-of-monitoring-methane-emissions-from-offshore-gas-production-and-transportation/.
Li, Z. et al. 2014. “Remote Sensing of Ecosystem Health: Opportunities, Challenges, and Future Perspectives.” Sensors. https://doi.org/10.3390/s141121117.
Liao, F. et al. n.d. MethaneSAT: A New Era of Transparency for Methane Measurement. Environmental Defense Fund. https://business.edf.org/wp-content/blogs.dir/90/files/MethaneSAT.pdf. Accessed Sept. 30, 2024.
Loeb, N. et al. 2021. “Satellite and Ocean Data Reveal Marked Increase in Earth’s Heating Rate.” Geophysical Research Letters. https://doi-org.proxy.library.upenn.edu/10.1029/2021GL093047.
Loew, A., et al. 2017. “Validation Practices for Satellite-based Earth Observation Data Across Communities.” Reviews of Geophysics. doi:10.1002/2017RG000562.
Loussaief, S. and A. Abdelkrim. 2016. “Machine Learning Framework for Image Classification.” 7th International Conference on Sciences of Electronics, Technologies of Information and Telecommunications (SETIT). doi: 10.1109/SETIT.2016.7939841.
Ma, Y., et al. 2015. “Remote Sensing Big Data Computing: Challenges and Opportunities.” Future Generation Computer Systems. doi.org/10.1016/j.future.2014.10.029.
Maganathan, T., et al. 2021. “Machine Learning and Data Analytics for Environmental Science: A Review, Prospects and Challenges.” 2020 IOP Conference Series: Material Science and Engineering. DOI 10.1088/1757-899X/955/1/012107.
McKenna, A., et al. 2019. The Role of Satellites and Smart Devices: Data Surprises and Security, Privacy, and Regulatory Challenges. Penn State Law Review. https://www.pennstatelawreview.org/wp-content/uploads/2019/06/Penn-StatimMcKenna-Formatted-FINAL.pdf.
MethaneSAT. 2023. “Data Sneak Peek: An Inside Look at What MethaneSAT will Measure.” https://www.methanesat.org/project-updates/data-sneak-peek-an-inside-look-at-what-methanesat-will-measure/.
MethaneSat. 2024. “New Data Show U.S. Oil and Gas Methane Emissions Over Four Times Higher than EPA Estimates.” https://www.methanesat.org/project-updates/new-data-show-us-oil-and-gas-methane-emissions-over-four-times-higher-epa-estimates.
NASA. n.d. “NISAR: NASA-ISRO SAR Mission.” https://nisar.jpl.nasa.gov/. Accessed Oct. 1, 2024.
NASA, n.d. “VISIONS: The EMIT Open Data Portal.” Last updated August 28, 2024. https://earth.jpl.nasa.gov/emit/data/data-portal/Greenhouse-Gases/. Accessed Oct. 1, 2024.
Nassar, A. and M. Kamal. 2021. “Ethical Dilemmas in AI-Powered Decision-Making: A Deep Dive into Big Data-Driven Ethical Considerations.” International Journal of Responsible Artificial Intelligence. https://neuralslate.com/index.php/Journal-of-Responsible-AI/article/view/43.
National Academies of Sciences, Engineering, and Medicine. 2022. Emissions Information for Decision Making: A Framework Going Forward. https://doi.org/10.17226/26641.
National Academies of Sciences, Engineering, and Medicine. 2022. Greenhouse Gas Emissions Information for Decision Making: A Framework Going Forward. https://doi.org/10.17226/26641.
National Ocean Service. n.d. “What Is Lidar?, https://oceanservice.noaa.gov/facts/lidar.html#:~:text=Lidar%2C%20which%20stands%20for%20Light,variable%20distances)%20to%20the%20Earth. Accessed Oct. 1, 2024.
NIST. 2024. “GReenhouse Gas And Air Pollutants Emissions System (GRA2PES).” Last updated Sept. 19, 2024. https://www.nist.gov/programs-projects/greenhouse-gas-and-air-pollutants-emissions-system-gra2pes. Accessed Sept. 30, 2024.
NOAA. n.d. “Global Monitoring Laboratory.” https://gml.noaa.gov/. Accessed Oct. 1, 2024.
Oda, S. and T. Inajima. 2024. “Japan Firms Unite to Cut Methane Emissions from LNG Supply.” Bloomberg, Oct. 4. https://www.bloomberg.com/news/articles/2024-10-04/japan-companies-unite-to-cut-methane-emissions-from-lng-supply.
Paddock, L. 2024. “The Critical Role of Advanced Monitoring in Environmental Compliance and Enforcement.” Environmental Law Institute, Sept. 5. https://www.eli.org/vibrant-environment-blog/critical-role-advanced-monitoring-environmental-compliance-and-enforcement.
Pennsylvania Department of Environmental Protection. 2023. Pennsylvania Greenhouse Gas Inventory Report 2023. https://files.dep.state.pa.us/Energy/Office%20of%20Energy%20and%20Technology/OETDPortalFiles/ClimateChange/FINAL_2023_GHG_Inventory_Report_2.27.24.pdf.
Pennsylvania Department of Environmental Protection. n.d. “The Principal Pollutants.” https://www.dep.pa.gov/Business/Air/BAQ/PollutantTopics/Pages/The-Principal-Pollutants.aspx. Accessed Sept. 30, 2024.
Perrone, P. et al. 2023. “The Relationship Between Spectral and Plant Diversity: Disentangling the Influence of Metrics and Habitat Types at the Landscape Scale.” Remote Sensing of Environment. https://doi.org/10.1016/j.rse.2023.113591.
Planet. n.d. https://www.planet.com/company/. Accessed Oct. 1, 2024.
Planet. 2024.“Planet’s Project Centinela: Monitoring Vulnerable Biodiversity Hotspots for Conservation Action.” September 30. https://www.planet.com/pulse/planets-project-centinela-monitoring-vulnerable-biodiversity-hotspots-for-conservation-action/.
Rajabi, R. et. Al. 2023. “Editorial: Hyperspectral Imaging in Environmental Monitoring and Analysis.” Frontiers in Environmental Science, Jan. 19. https://doi.org/10.3389/fenvs.2023.1353447.
Ravikumar, A. n.d. “Fugitive Emissions Abatement Simulation Toolkit.” https://www.arvindravikumar.com/feast. Accessed Oct. 3, 2024.
Testbook. 2023. “Remote Sensing: Definition, Components, Types, Principles and Uses.” Last updated on May 2, 2023. https://testbook.com/physics/remote-sensing.
Salamone, F. et al. 2021. “Wearable Devices for Environmental Monitoring in the Built Environment: A Systematic Review.” Sensors. https://doi.org/10.3390/s21144727.
Schneider, T. et al. 2023. “Harnessing AI and Computing to Advance Climate Modelling and Prediction.” Nature Climate Change. https://doi.org/10.1038/s41558-023-01769-3.
Shah, J. and B. Mishra. 2016. “IoT Enabled Environmental Monitoring System for Smart Cities.” IOTA Programming System. doi: 10.1109/IOTA.2016.7562757.
Sherwin, E. et al. 2024. “US Oil and Gas System Emissions from Nearly One Million Aerial Site Measurements. Nature. https://doi.org/10.1038/s41586-024-07117-5.
SkyTruth. n.d. https://skytruth.org/. Accessed Oct. 1, 2024.
Synoptic. n.d. https://synopticdata.com/. Accessed Oct. 1, 2024.
TEMPO. 2024 “A Global Constellation of Satellites Aimed at Measuring Air Quality.” Feb. 14. https://tempo.si.edu/blog/2023_Feb_14.html.
Thompson, D., et. al. 2018. “Optimal Estimation for Imaging Spectrometer Atmospheric Correction.” Remote Sensing of Environment. https://doi.org/10.1016/j.rse.2018.07.003.
Turner, W., et al. 2015. “Free and Open-access Satellite Data Are Key to Biodiversity Conservation.” Biological Conservation. https://doi.org/10.1016/j.biocon.2014.11.048.
Umar, A. 2022. “A Digital Transformation Lab for Developing Countries and Small to Medium Enterprises.” 2022 IEEE European Technology and Engineering Management Summit (E-TEMS). doi: 10.1109/E-TEMS53558.2022.9944423.
U.N. Environment Programme. n.d. ‘‘Global Environment Monitoring.’’ https://wesr.unep.org/article/global-environment-monitoring. Accessed Oct. 1, 2024.
U.N. Environment Programme. n.d. Methane Alert and Response System (MARS). ” https://www.unep.org/explore-topics/energy/what-we-do/methane/imeo-action/methane-alert-and-response-system-mars. Accessed Sept. 30, 2024.
U.N. Environment Programme. 2021. “Methane Emissions Are Driving Climate Change. Here’s How to Reduce Them.” Aug. 20. https://www.unep.org/news-and-stories/story/methane-emissions-are-driving-climate-change-heres-how-reduce-them.
U.S. Department of Energy. 2024. “Greenhouse Gas Supply Chain Emissions Measurement, Monitoring, Reporting, Verification Framework.” Last updated on October 15, 2024. https://www.energy.gov/fecm/greenhouse-gas-supply-chain-emissions-measurement-monitoring-reporting-verification-framework.
U.S. Energy Information Administration. 2024. “The United States Remained the Largest Liquefied Natural Gas Supplier to Europe in 2023.” Feb. 29. https://www.eia.gov/todayinenergy/detail.php?id=61483.
UN Environment. 2019. “Global Environment Outlook – GEO-6: Healthy Planet, Healthy People.” https://wedocs.unep.org/20.500.11822/27539.
U.S. Environmental Protection Agency. 2024. Air Monitoring for Fine Particle Pollution (PM2.5) Fact Sheet. https://www.epa.gov/system/files/documents/2024-02/pm-naaqs-monitoring-fact-sheet.pdf
U.S. Environmental Protection Agency. n.d. “AirNow.” https://www.airnow.gov/. Accessed Sept. 30, 2024.
U.S. Environmental Protection Agency, 2024. “Cumulative Impacts Research.” Last updated on May 23, 2024. https://www.epa.gov/healthresearch/cumulative-impacts-research.
U.S. Environmental Protection Agency. 2024. “Data.” Last updated on October 4, 2024. https://www.epa.gov/data.
U.S. Environmental Protection Agency. 2024. “National Emissions Inventory (NEI).” Last updated on May 6, 2024. https://www.epa.gov/air-emissions-inventories/national-emissions-inventory-nei.
U.S. Environmental Protection Agency. 2024. “Rulemaking Notices for GHG Reporting.” Last updated on November 13, 2024. https://www.epa.gov/ghgreporting/rulemaking-notices-ghg-reporting.
U.S. Environmental Protection Agency, “Waste Emissions Charge.” Last updated on November 12, 2024. https://www.epa.gov/inflation-reduction-act/waste-emissions-charge.
U.S. Environmental Protection Agency. 2020. “What Are Cumulative Impacts?” July 7. https://usepa.servicenowservices.com/ecss/en/what-are-cumulative-impacts?id=kb_article_view&sys_kb_id=36ce5ba21bb99c1013bdb913cc4bcbc1.
U.S. Greenhouse Gas Center, 2024. https://earth.gov/ghgcenter. Accessed Oct. 8, 2024.
Wettengel, J. 2024. “Germany, EU Remain Heavily Dependent on Imported Fossil Fuels.” Clean Energy Wire, April 3, https://www.cleanenergywire.org/factsheets/germanys-dependence-imported-fossil-fuels.
Willard, J., et al. 2023. “Integrating Scientific Knowledge with Machine Learning for Engineering and Environmental Systems.” ACM Computer Surveys. https://doi.org/10.1145/3514228.
World Economic Forum. 2024. Global Risks Report, 2024. https://www3.weforum.org/docs/WEF_The_Global_Risks_Report_2024.pdf
York, N. et al. 2023 “Justice and Ethics in Conservation Remote Sensing: Current Discourses and Research Needs.” Biological Conservation, doi.org/10.1016/j.biocon.2023.110319.
Zhang, S. et al. 2023. “Atmospheric Remote Sensing for Anthropogenic Methane Emissions: Applications and Research Opportunities.” Science of The Total Environment. https://doi.org/10.1016/j.scitotenv.2023.164701.
Zhao, Q. et al. 2022. “An Overview of the Applications of Earth Observation Satellite Data: Impacts and Future Trends.” Remote Sensing. https://doi.org/10.3390/rs14081863.
Zhu, Y et al. 2024. “Geospatial Life Cycle Analysis of Greenhouse Gas Emissions from US Liquefied Natural Gas Supply Chains.” ChemRxiv. doi:10.26434/chemrxiv-2024-9v8dw. This content is a preprint and has not been peer-reviewed.
- An example of this is that in Pennsylvania, in addition to the Pennsylvania Department of Environmental Protection, local air pollution control agencies in Philadelphia and Allegheny counties monitor air pollution. [↩]
- The U.S. Environmental Protection Agency (EPA) primarily focuses its regulatory efforts on “major sources” of air pollution – larger industrial facilities that have the potential to emit 100 tons per year or more of any criteria air pollutant or precursor. Regulation of smaller facilities if left to states and local agencies, and approaches vary widely. [↩]
- See MethaneSAT, Data Sneak Peek: An Inside Look at What MethaneSAT will Measure, https://www.methanesat.org/project-updates/data-sneak-peek-an-inside-look-at-what-methanesat-will-measure/, accessed Sept. 30, 2024. [↩]
- While access to some types of data from government monitoring systems has historically been restricted to protect corporate information or national security, much of the data that U.S. EPA collects is in the public domain See https://www.epa.gov/data (accessed Oct. 1, 2024). Data from monitoring systems described in this paper are intended to be extensions of and additions to that body of publicly available data. [↩]
- Machine learning and satellite imagery have, for example, been used to map industrial infrastructure at sea — from fishing vessels to wind turbines. The findings provide a more comprehensive picture of maritime activity than ever before. The same intelligence-gathering approach should be applied to compliance monitoring. See Klemmer and Rolf 2024. [↩]
- National Environmental Policy Act (NEPA), Clean Air Act (CAA), Clean Water Act (CWA): Resource Conservation and Recovery Act (RCRA), Comprehensive Environmental Response, Compensation, and Liability Act (CERCLA or Superfund), and Endangered Species Act (ESA). [↩]